Protecting group: Difference between revisions
→Carbonyl protecting groups: Most stays, it turns out |
→Carboxylic acid protecting groups: Copy before edit |
||
Line 162: | Line 162: | ||
===Carboxylic acid protecting groups=== |
===Carboxylic acid protecting groups=== |
||
The most important protecting groups for [[Carboxylic acid|carboxylic acids]] are the esters of various alcohols. There next-most-used are the ortho-esters and [[oxazoline]], but of much lesser importance. For the formation of carboxy-esters there are a wide variety of methods:<ref>P.J. Kocieński: ''Protecting Groups'', pp. 119.</ref> |
|||
* Direct esterification of a carboxylic acids and an alcoholic compound. Because of the adverse reaction-equilibrium balance between alcohols and carboxylic acids, the equlibrium must be transformed via either removal of water or a workup with a great excess of alcohol. Therefore the alcohol must be absolutely quite cheap. This reaction is acid-catalyzed (sulfuric acid, ''p''-toluenesulfonic acid or acid ion-exchange media are the typical trans-esterifiaction catalysts). |
|||
* The reaction of acid anhydrides or chlorides with alcohols in the presence of an auxiliary base. For the base co-reactant one finds commonly [[pyridine]], [[diisopropylethylamine]] or [[triethylamine]] applied. These reactions can be catalyzed with [[4-(Dimethylamino)pyridine|4{{nbh}}''N'',''N''{{nbh}}dimethylamino­pyridine]], which raises the reaction rate relative to pure pyridine by a factor of 10<sup>4</sup>. Compared to the direct esterification procedure these methods are under quite mild conditions. |
|||
* The reaction of carboxylic acid salts with alkyl halides is another method to form carboxylic acid esters. |
|||
* The reaction of carboxylic acids with [[isobutene]] is a gentle method to form ''tert''{{nbh}}butyl esters. Here isobutene and the carboxylic acid react in the presence of a strong acid like [[sulfuric acid]]. |
|||
* The reaction of carboxylic acids with [[Diazoalkane|diazoalkanes]] is a very gentle and quantitative method to form esters. It is used primarily for the formation of methyl and benzyl esters on account of the inaccesibility of compex diazoalkanes. |
|||
Besides these classical methods for esterification, other modern techniques have been developed for special reactions. |
|||
* The [[Carboxylic acid activation|activation of carboxylic acids]] with [[dicyclohexylcarbodiimide]] and reaction of the so-formed ''O''{{nbh}}acyl­isoureas with the alcoholic compound in the presence of 4{{nbh}}''N'',''N''{{nbh}}dimethylamino­pyridine (the [[Steglich esterification]]). |
|||
* Activation of a carboxylic acid with formation of a mixed anhydride with [[2,4,6-trichlorobenzoic acid]] via reaction of the carboxylic acid with benzoyl chloride in the presence of 4{{nbh}}''N'',''N''{{nbh}}dimethylamino­pyridine and triethylamine. The mixed anhydride is formed ''in situ'' and immediately reacted with the alcoholic compound ([[Yamaguchi esterification]]). |
|||
* Activation of the alcoholic compound via reaction under [[Mitsunobu reaction|Mitsunobu conditions]] with [[diethylazodicarboxylate]] and [[triphenylphosphine]] and associated reaction ''in situ'' with the carboxylic acid ([[Mitsunobu reaktion|Mitsunobu esterification]]). |
|||
Many groups can suffice for the alcoholic component. Here the methyl, ''tert''-butyl, benzyl, and allyl esters are very commonly used. Moreover, a whole family of protecting groups are formed therefrom, which derive from the ester protection of the hydroxy group. The specific cleaving conditions are contrariwise generally quite similar. Basically, each ester can be hydrolyzed in the presence of hydroxide ions in a mixed water-alcohol solution. For sensitive substrates, it is typically appropriate to apply [[lithium hydroxide]] in tetrahydrofuran and methanol. From the hydrolytic tendencies the same rules naturally apply to esters as a protecting group for alcohols. |
|||
{| class="wikitable" |
|||
!Name |
|||
!Formula |
|||
!Abbrev. |
|||
!Cleavage |
|||
!Special formation {{sic|?}} |
|||
|- |
|||
|Methyl |
|||
|[[File:Me_is.svg|80x80px]] |
|||
|Me |
|||
|Nucleophilic/alkali with a metal hydroxide or carbonate in wet methanol or tetrahydrofuran,<ref>Satomi Niwayama: „Highly Efficient Selective Monohydrolysis of Symmetric Diesters“, in: ''[[J. Org. Chem.]]'', '''2000''', ''65'', pp. 5834–5836; [[doi:10.1021/jo0001986]].</ref><ref>J.M. Khurana, Arti Sehgal: „An efficent and convenient procedure for ester hydrolysis“, in: ''[[Org. Prep. Proced. Ind.]]'', '''1994''', ''26'', pp. 580–583.</ref> alkali-halogen salts in wet aprotic solutions like dimethyl sulfoxide or heated ''N'',''N''{{nbh}}dimethylformamide,<ref>Robert V. Stevens, Albert W. M. Lee: „Stereochemistry of the Robinson-Schoepf reaction. A stereospecific total synthesis of the ladybug defense alkaloids precoccinelline and coccinelline“, in: ''[[J. Am. Chem. Soc.]]'', '''1979''', ''101'', pp. 7032–7035; [[doi:10.1021/ja00517a042]].</ref><ref>J. Wrobel, K. Takahashi, V. Honkan, G. Lannoye, J. M. Cook, Steven H. Bertz: „α-Lithio ketones. 1. Stereocontrolled synthesis of (±)-modhephene via the Weiss reaction“, in: ''[[J. Org. Chem.]]'', '''1983''', ''48'', pp. 139–141; [[doi:10.1021/jo00149a034]].</ref><ref>Dennis D. Keith, John A. Tortora, Roxana Yang: „Synthesis of L-2-amino-4-methoxy-trans-but-3-enoic acid“, in: ''[[J. Org. Chem.]]'', '''1978''', ''43'', pp. 3711–3713; [[doi:10.1021/jo00413a016]].</ref> or enzymatic, i. e. with [[pig liver esterase]]<ref>Peter Mohr, Nada Waespe-Šarčević, Christoph Tamm, Krystyna Gawronska, Jacek K. Gawronski: „A Study of Stereoselective Hydrolysis of Symmetrical Diesters with Pig Liver Esterase“, in: ''[[Helv. Chim. Acta]]'', '''1983''', ''66'', pp. 2501–2511; [[doi:10.1002/hlca.19830660815]].</ref><ref>Théophile Tschamber, Nada Waespe-Šarčević, Christoph Tamm: „Stereocontrolled Synthesis of an Epimer of the C(19)-to-C(27) Segment of Rifamycin S“, in: ''[[Helv. Chim. Acta]]'', '''1986''', ''69'', pp. 621–625; [[doi:10.1002/hlca.19860690311]].</ref> |
|||
|Diazomethane in diethyl ether,<ref>Yves Rubin, Carolyn B. Knobler, Francois Diederich: „Precursors to the cyclo[n]carbons: from 3,4-dialkynyl-3-cyclobutene-1,2-diones and 3,4-dialkynyl-3-cyclobutene-1,2-diols to cyclobutenodehydroannulenes and higher oxides of carbon“, in: ''[[J. Am. Chem. Soc.]]'', '''1990''', ''112'', pp. 1607–1617; [[doi:10.1021/ja00160a047]].</ref><ref>Sunggak Kim, Yong Gil Kim, Deog-il Kim: „A novel method for selective dioxolanation of ketones in the presence of aldehydes“, in: ''[[Tetrahedron Lett.]]'', '''1992''', ''33'', pp. 2565–2566; [[doi:10.1016/S0040-4039(00)92243-3]].</ref><ref>G. Bauduin, D. Bondon, Y. Pietrasanta, B. Pucci: „Reactions de transcetalisation – II: Influence des facteurs steriques et electroniques sur les energies de cetalisation“, in: ''[[Tetrahedron]]'', '''1978''', ''34'', pp. 3269–3274; [[doi:10.1016/0040-4020(78)80243-9]].</ref> [[caesium carbonate]] and methyl iodide in ''N'',''N''{{nbh}}Dimethylformamide,<ref>John E. McMurry, Stephen J. Isser: „Total synthesis of longifolene“, in: ''[[J. Am. Chem. Soc.]]'', '''1972''', ''94'', pp. 7132–7137; [[doi:10.1021/ja00775a044]].</ref> or methanol and catalytic trimethylsilyl chloride<ref>M.P. Bosch, M. Pilar Bosch, Francisco Camps, Jose Coll, Angel Guerrero, Toshio Tatsuoka, Jerrold Meinwald: „A stereoselective total synthesis of (±)-muzigadial“, in: ''[[J. Org. Chem.]]'', '''1986''', ''51'', pp. 773–784; [[doi:10.1021/jo00356a002]].</ref> |
|||
|- |
|||
|''tert''{{nbh}}Butyl |
|||
|[[File:TBu_is.svg|100x100px]] |
|||
|''tert''{{nbh}}Bu |
|||
|acid: trifluoroacetic acid (pure or in dichloromethane),<ref>Ulrich Schmidt, Thomas Beuttler, Albrecht Lieberknecht, Helmut Griesser: „Aminosäuren und peptide – XXXXII. Synthese von Chlamydocin + epi-Chlamydocin“, in: ''[[Tetrahedron Lett.]]'', '''1983''', ''24'', pp. 3573–3576; [[doi:10.1016/S0040-4039(00)88171-X]].</ref> formic acid, or ''p''{{nbh}}toluenesulfonic acid<ref>Elias J. Corey, Plato A. Magriotis: „Total synthesis and absolute configuration of 7,20-diisocyanoadociane“, in: ''[[J. Am. Chem. Soc.]]'', '''1987''', ''109'', pp. 287–289; [[doi:10.1021/ja00235a052]].</ref> |
|||
|Isobutene in dioxane and catalytic sulfuric acid<ref>Elias J. Corey, Kyriacos C. Nicolaou, Takeshi Toru: „Total synthesis of (±)-vermiculine“, in: ''[[J. Am. Chem. Soc.]]'', '''1975''', ''97'', pp. 2287–2288; [[doi:10.1021/ja00841a058]].</ref><ref>Tainejiro Hiyama, Akihiro Kanakura, Hajime Yamamoto, Hitosi Nozaki: „General Route to α,β-unsaturated Aldehydes of Homoterpenoid and terpenoid Structure. Sythesis of JH-II and β-Sinensal“, in: ''[[Tetrahedron Lett.]]'', '''1978''', ''19'', pp. 3051–3054; [[doi:10.1016/S0040-4039(01)94936-6]].</ref><ref>F. Huet, A. Lechevallier, M. Pellet, J.M. Conia: „Wet Silica Gel; A Convenient Reagent for Deacetalization“, in: ''[[Synthesis]]'', '''1978''', pp. 63–64.</ref> |
|||
|- |
|||
|Benzyl |
|||
|[[File:Bn_is.svg|100x100px]] |
|||
|Bn |
|||
|Hydrogenolytic: hydrogen/palladium on activated carbon<ref>F. Zymalkokowski: ''Katalytische Hydrierung'', Ferdinand Enke Verlag, Stuttgart 1965, pp. 127–133.</ref> |
|||
| |
|||
|- |
|||
|[[Diphenylmethylester|Benzhydryl]] |
|||
|[[File:Benzhydrol_is.svg|117x117px]] |
|||
| |
|||
|hydrogenolytic: hydrogen/palladium on activated carbon (very easy to cleave)<ref>P.J. Kocieński: ''Protecting Groups'', pp. 136.</ref> |
|||
| |
|||
|- |
|||
|Allyl |
|||
|[[File:Allyl_is.svg|100x100px]] |
|||
|Allyl |
|||
|Similar to ethers: potassium ''tert''{{nbh}}but­oxide, palladium on activated carbon, [[DABCO]] (1,4{{nbh}}diaza­bicyclo[2.2.2]octane) in methanol, diverse platinum complexes – connected with acid workup<ref>P.J. Kocieński: ''Protecting Groups'', pp. 139–142.</ref> |
|||
| |
|||
|} |
|||
Protection of [[carboxylic acid]]s: |
Protection of [[carboxylic acid]]s: |
||
* [[Methyl]] [[esters]] – Removed by acid or base. |
* [[Methyl]] [[esters]] – Removed by acid or base. |
Revision as of 21:11, 28 February 2024

A protecting group or protective group is introduced into a molecule by chemical modification of a functional group to obtain chemoselectivity in a subsequent chemical reaction. It plays an important role in multistep organic synthesis.[1]
In many preparations of delicate organic compounds, specific parts of the molecules cannot survive required reagents or chemical environments. These parts (functional groups) must be protected. For example, lithium aluminium hydride is a highly reactive reagent that usefully reduces esters to alcohols. It always reacts with carbonyl groups, and cannot be discouraged by any means. When an ester must be reduced in the presence of a carbonyl, hydride attack on the carbonyl must be prevented. One way to do so converts the carbonyl into an acetal, which does not react with hydrides. The acetal is then called a protecting group for the carbonyl. After the hydride step is complete, aqueous acid removes the acetal, restoring the carbonyl. This step is called deprotection.
Protecting groups are more common in small-scale laboratory work and initial development than in industrial production because they add additional steps and material costs. However, compounds with repetitive functional groups – generally, biomolecules like peptides, oligosaccharides or nucleotides – may require protecting groups to order their assembly. Also, cheap chiral protecting groups may often shorten an enantioselective synthesis (e.g. shikimic acid for oseltamivir).
Orthogonal protection

Orthogonal protection is a strategy allowing the specific deprotection of one protective group in a multiply-protected structure. For example, the amino acid tyrosine could be protected as a benzyl ester on the carboxyl group, a fluorenylmethylenoxy carbamate on the amine group, and a tert-butyl ether on the phenol group. The benzyl ester can be removed by hydrogenolysis, the fluorenylmethylenoxy group (Fmoc) by bases (such as piperidine), and the phenolic tert-butyl ether cleaved with acids (e.g. with trifluoroacetic acid).
A common example for this application, the Fmoc peptide synthesis, in which peptides are grown in solution and on solid phase, is very important.[2] The protecting groups in solid-phase synthesis with regard to the reaction conditions such as reaction time, temperature and reagents can be standardized so that they are carried out by a machine, while yields of well over 99% can be achieved. Otherwise, the separation of the resulting mixture of reaction products is virtually impossible.[3]
-
Schematic diagram of a solid-state peptide synthesis with orthogonal protecting groups X and Y
-
Fmoc solid state peptide synthesis with orthogonal protecting groups
The technique was introduced in the field of peptide synthesis by Robert Bruce Merrifield in 1977.[4]
A further important example of orthogonal protecting groups occurs in carbohydrate chemistry. As carbohydrates or hydroxyl groups exhibit very similar reactivities, a transformation that protects or deprotects a single hydroxy group must be possible for a successful synthesis.
Cleavage categorization
Many reaction conditions have been established, under which, in accordance with the concept of orthogonality, some protecting groups cleave. One can roughly distinguish between the following environments:[5]
- Acid-labile protecting groups
- Base-labile protecting groups
- Fluoride-labile protecting groups
- Enzyme-labile protecting groups
- Reduction-labile protecting groups
- Oxidation-labile protecting groups
- Protecting groups cleaved by heavy metal salts or their complexes.
- Photo-labile protecting groups
- Double-layered protecting groups
A wide variety of groups are cleaved in acid or base conditions, but the others are more unusual.
Flouride ions form very strong bonds to silicon; thus silicon protecting groups are almost invariably removed by fluoride ions. Each type of counterion, i.e. cleavage reagent, can also selectively cleave different silicon protecting groups depending on steric hindrance. The advantage of fluoride-labile protecting groups is that no other protecting group is attacked by the cleavage conditions.
Lipases and other enzymes cleave ethers at biological pH (5-9) and temperatures (30–40 °C). Because enzymes have very high substrate specificity, the method is quite rare, but extremely attractive.
Catalytic hydrogenation removes a wide variety of benzyl groups: ethers, esters, urethanes, carbonates, etc.
Only a few protecting groups can be detached oxidatively: the methoxybenzyl ethers, which oxidize to a quinomethide. They can be removed with ceric ammonium nitrate (CAN) or dichlorodicyanobenzoquinone (DDQ).
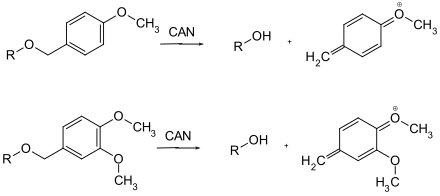

Allyl compounds will isomerize to a vinyl group in the presence of noble metals. The residual enol ether (from a protected alcohol) or enamine (resp. amine) hydrolyzes in light acid.
Photolabile protecting groups bear a chromophore, which is activated through radiation with an appropriate wavelength and so can be removed.[6] For examples the o-nitrobenzylgroup ought be listed here.
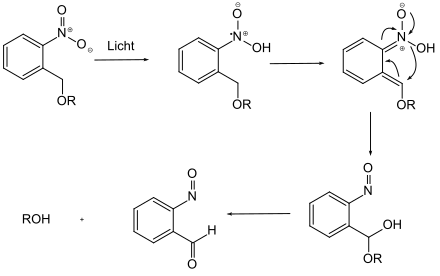
The rare double-layer protecting group is a protected protecting group, which exemplify high stability.
Common protecting groups
Alcohol protecting groups
The classical protecting groups for alcohols are esters, deprotected by nucleophiles; triorganosilyl ethers, deprotected by acids and fluoride ions; and (hemi)acetals, deprotected by weak acids. In rarer cases, a carbon ether might be used.
The most important esters with common protecting-group use are the acetate, benzoate, and pivalate esters, for these exhibit differential removal. Sterically hindered esters are less susceptible to nucleophilic attack:
- Chloroacetyl > acetyl > benzoyl > pivaloyl

Triorganosilyl sources have quite variable prices, and the most economical is chlorotrimethylsilane (TMS-Cl), a Direct Process byproduct. The trimethylsilyl ethers are also extremely sensitive to acid hydrolysis (for example silica gel suffices as a proton donator) and are consequently rarely used nowadays as protecting groups.
Aliphatic methyl ethers cleave with difficulty and only under drastic conditions, so that these are in general only used with quinonic phenols. However, hemiacetals and acetals are much easier to cleave.

List
Esters:
- Acetyl (Ac) – Removed by acid or base (see Acetoxy group).
- Benzoyl (Bz) – Removed by acid or base, more stable than Ac group.
- Pivaloyl (Piv) – Removed by acid, base or reductant agents. It is substantially more stable than other acyl protecting groups.
Silyl ethers:
- Trimethylsilyl (TMS) — Potassium fluoride, acetic acid or potassium carbonate in methanol[7]
- Triethylsilyl — 10–100× stabler than a TMS group.[8] Cleaved with trifluoroacetic acid in water/tetrahydrofuran,[9] acetic acid in water/tetrahydrofuran,[10] or hydrogen fluoride in water or pyridine[11]
- tert-Butyldimethylsilyl (TBDMS or TBS) — Cleaved with acetic acid in tetrahydrofuran/water,[12] Pyridinium tosylate in methanol,[13] trifluoroacetic acid in water,[14] hydrofluoric acid in acetonitrile,[15] pyridinium fluoride in tetrahydrofuran,[16] tetrabutylammonium fluoride in THF.[17] Commonly protects 2'-hydroxy function in oligonucleotide synthesis.
- Triisopropylsilyl (TIPS) ethers) — Similar conditions to TBS but longer reaction times.[18]
- tert‑Butyldiphenylsilyl (TBDPS) — Similar conditions to TBS but even longer reaction times (100–250× slower than TBS and 5–10× slower than TIPS)
Benzyl ethers:
- Benzyl (Bn) — Removed by hydrogenolysis.[19] Bn group is widely used in sugar and nucleoside chemistry.
- Trityl (triphenylmethyl, Tr) — Removed by acid[20][21][22] and hydrogenolysis
- p-Methoxybenzyl ether (PMB) — Removed by acid, hydrogenolysis, or oxidation – commonly with DDQ.[23]
- p,m‑Dimethoxybenzyl ether — Removed via oxidation with DDQ or ceric ammonium chloride[24]
Acetals:
- Dimethoxytrityl, [bis-(4-methoxyphenyl)phenylmethyl] (DMT) — Removed by weak acid. DMT group is widely used for protection of 5'-hydroxy group in nucleosides, particularly in oligonucleotide synthesis.
- Methoxytrityl [(4-methoxyphenyl)diphenylmethyl] (MMT) – Removed by acid and hydrogenolysis.
- Benzyloxymethyl — Comparable stability to MOM, MEM und SEM,[25] but also admits reductive removal: sodium in liquid ammonia,[26][27] catalytic hydrogenation (palladium hydroxide on activated carbon), or Raney nickel in ethanol[28][29]
- Ethoxyethyl ethers (EE) – Cleavage more trivial than simple ethers e.g. 1N hydrochloric acid[30]
- Methoxyethoxymethyl ether (MEM) — Removed by hydrobromic acid in tetrahydrofuran[31] or zinc bromide in dichloromethane[32]
- Methoxymethyl ether (MOM) — Removed by 6 M hydrochloric acid in tetrahydrofuran/water[33]
- Tetrahydropyranyl (THP) — Removed by acetic acid in tetrahydrofuran/water,[34] p‑toluenesulfonic acid in methanol[35]
- Methylthiomethyl ether — Removed by acid[citation needed] or soft metal oxidants: base-buffered mercuric chloride in wet acetonitrile[36] or silver nitrate in wet tetrahydrofuran[37]
- Tris(isopropyl)silyloxymethyl (TOM) — Commonly protects 2'-hydroxy function in oligonucleotide synthesis.
- β‑(Trimethylsilyl)ethoxymethyl — More labile than MEM and MOM to acid hydrolysis: 0.1 M hydrochloric acid in methanol,[38] concentrated hydrofluoric acid in acetonitrile,[39] boron trifluoride etherate in dichloromethane,[40] or tetrabutylammonium fluoride in HMPT (Hexamethyl phosphoric acid triamide) or in tetrahydrofuran[41][42]
Other ethers:
- p-Methoxyphenyl ether (PMP) – Removed by oxidation.[citation needed]
- Tert-butyl ethers (tBu) – Removed with anhydrous trifluoroacetic acid, hydrogen bromide in acetic acid, or 4 N hydrochloric acid[43]
- Allyl — Removed with potassium tert‑butoxide[44] DABCO in methanol, palladium on activated carbon, or diverse platinum complexes – conjoined with acid workup.[45]
- Methyl ethers – Cleavage is by TMSI in dichloromethane or acetonitrile or chloroform. An alternative method to cleave methyl ethers is BBr3 in DCM
- Tetrahydrofuran (THF)[clarification needed] – Removed by acid.
1,2-Diols
The 1,2‑diols (glycols) present for protecting-group chemistry a special class of alcohols. One can exploit the adjacency of two hydroxy groups, e.g. in sugars, in that one protects both hydroxy groups codependently as an acetal. Common in this situation are the benzylidene, isopropylidene and cyclohexylidene or cyclopentylidene acetals.

An exceptional case appears with the benzylideneprotecting group,which also admits reductive cleavage. This proceeds either through catalytic hydrogenation or with the hydride donor diisobutyl aluminum hydride (DIBAL). The cleavage with DIBAL deprotects one alcohol group, for the benzyl moiety stays as a benzyl ether on the second, sterically hindered hydroxy group.[46][47]

Amine protecting groups

Amines have a special importance in peptide synthesis, but are a quite potent nucleophile and also relatively strong bases. These characteristics imply that new protecting groups for amines are always under development.[48]
Amine groups are primarily protected through acylation, typically as a carbamate. When a carbamate deprotects, it evolves carbon dioxide. The commonest-used carbamates are the tert-butoxycarbonyl, benzoxycarbonyl, fluorenylmethylenoxycarbonyl, and allyloxycarbonyl compounds.
Other, more exotic amine protectors are the phthalimides, which admit reductive cleavage,[49] and the trifluoroacetamides, which hydrolyze easily in base. Indoles, pyrroles und imidazoles — verily any aza-heterocycle — admit protection as N‑sulfonylamides,which are far too stable with aliphatic amines.[50] N‑benzylated amines can be removed through catalytic hydrogenation or Birch reduction, but have a decided drawback relative to the carbamates or amides: they retain a basic nitrogen.
List
Carbamates:
- Carbamate group – Removed by acid and mild heating.
- Carbobenzyloxy (Cbz) group — Removed by hydrogenolysis: hydrogen and palladium on activated carbon,[51] or lithium or sodium in liquid ammonia.[52]
- p-Methoxybenzyloxycarbonyl (Moz or MeOZ) group – Removed by hydrogenolysis, more labile than Cbz
- tert-Butyloxycarbonyl (Boc) group — Removed by concentrated strong acid (such as HCl[53] or CF3COOH[54]), or by heating to >80 °C. Common in solid phase peptide synthesis.
- 9-Fluorenylmethyloxycarbonyl (Fmoc) group — Removed by base, such as 20–50 % piperidine in dimethylformamide (DMF)[55] or N-Methyl-2-pyrrolidone,[56], or 50 % morpholine in DMF for sensitive glycopeptides.[57][58] Common in solid phase peptide synthesis
- Allyloxycarbamate group — Removed with complexes of metals like palladium(0) or nickel(0).[59]
Other amides:
- Acetyl (Ac) group is common in oligonucleotide synthesis for protection of N4 in cytosine and N6 in adenine nucleic bases and is removed by treatment with a base, most often, with aqueous or gaseous ammonia or methylamine. Ac is too stable to be readily removed from aliphatic amides.
- Benzoyl (Bz) group is common in oligonucleotide synthesis for protection of N4 in cytosine and N6 in adenine nucleic bases and is removed by treatment with a base, most often with aqueous or gaseous ammonia or methylamine. Bz is too stable to be readily removed from aliphatic amides.
- Troc (trichloroethyl chloroformate ) group – Removed by Zn insertion in the presence of acetic acid
- Tosyl (Ts) group – Removed by concentrated acid (HBr, H2SO4) & strong reducing agents (sodium in liquid ammonia or sodium naphthalenide)
- Other sulfonamide (Nosyl & Nps) groups – Removed by samarium iodide, thiophenol or other soft thiol nucleophiles, or tributyltin hydride[60]
Benzylamines:
- Benzyl (Bn) group – Removed by hydrogenolysis
- p-Methoxybenzyl (PMB) – Removed by hydrogenolysis, more labile than benzyl
- 3,4-Dimethoxybenzyl (DMPM) – Removed by hydrogenolysis, more labile than p-methoxybenzyl
- p-Methoxyphenyl (PMP) group – Removed by ammonium cerium(IV) nitrate (CAN)
Carbonyl protecting groups
The most common protecting groups for carbonyls are acetals and typically cyclic acetals with diols. The runners-up used are also cyclic acetals with 1,2‑hydroxythiols or dithioglycols – the so-called O,S– or S,S-acetals.


Overall, trans-acetalation plays a lesser role in forming protective acetals; they are formed as a rule from glycols through dehydration. Normally a simple glycol like ethylene glycol or 1,3-propadiol is used for acetalation.Modern variants also use glycols, but with the hydroxyl hydrogens replaced with a trimethylsilyl group.[61][62]
Acetals can be removed in acidic aqueous conditions. For those ends, the mineral acids are appropriate acids. Acetone is a common cosolvent, used to promote dissolution. For a non-acidic cleavage technique, a palladium(II) chloride acetonitrile complex in acetone[63] or iron(III) chloride on silica gel can be performed with workup in chloroform.[64]
Cyclic acetals are very much more stable against acid hydrolysis than acyclic acetals. Consequently acyclic acetals are used practically only when a very mild cleavage is required or when two different protected carbonyl groups must be differentiated in their liberation.[65]
Besides the O,O-acetals, the S,O- and S,S-acetals also have an application, albeit scant, as carbonyl protecting groups too. Thiols, which one begins with to form these acetals, have a very unpleasant stench and are poisonous, which severely limit their applications. Thioacetals and the mixed S,O-acetals are, unlike the pure O,O-acetals, very much stabler against acid hydrolysis. This enables the selective cleavage of the latter in the presence of sulfur-protected carbonyl groups.
The formation of S,S-acetals normally follows analogously to the O,O-acetals with acid catalysis from a dithiol and the carbonyl compound. Because of the greater stability of thioacetals, the equilibirum lies on the side of the acetal. In contradistinction to the O,O‑acetal case, it is not needed to remove water from the reaction mixture in order to shift the equilibrium.[66]
S,O-Acetals are hydrolyzed a factor of 10,000 times faster than the corresponding S,S-acetals. Their formation follows analogously from the thioalcohol. Also their cleavage proceeds under similar conditions and predominantly through mercury(II) compounds in wet acetonitrile.[67]
For aldehydes, a temporary protection of the carbonyl group the presence of ketones as hemiaminal ions is shown below. Here it is applied, that aldehydes are very much more activated carbonyls than ketones and that many addition reactions are reversible.[68][69]
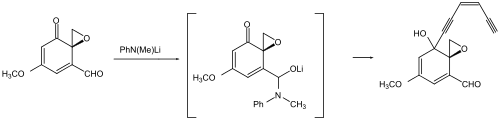
Types of protectants
- Acetals and Ketals – Removed by acid. Normally, the cleavage of acyclic acetals is easier than of cyclic acetals.
- Acylals – Removed by Lewis acids.
- Dithianes – Removed by metal salts or oxidizing agents.
Carboxylic acid protecting groups
The most important protecting groups for carboxylic acids are the esters of various alcohols. There next-most-used are the ortho-esters and oxazoline, but of much lesser importance. For the formation of carboxy-esters there are a wide variety of methods:[70]
- Direct esterification of a carboxylic acids and an alcoholic compound. Because of the adverse reaction-equilibrium balance between alcohols and carboxylic acids, the equlibrium must be transformed via either removal of water or a workup with a great excess of alcohol. Therefore the alcohol must be absolutely quite cheap. This reaction is acid-catalyzed (sulfuric acid, p-toluenesulfonic acid or acid ion-exchange media are the typical trans-esterifiaction catalysts).
- The reaction of acid anhydrides or chlorides with alcohols in the presence of an auxiliary base. For the base co-reactant one finds commonly pyridine, diisopropylethylamine or triethylamine applied. These reactions can be catalyzed with 4‑N,N‑dimethylaminopyridine, which raises the reaction rate relative to pure pyridine by a factor of 104. Compared to the direct esterification procedure these methods are under quite mild conditions.
- The reaction of carboxylic acid salts with alkyl halides is another method to form carboxylic acid esters.
- The reaction of carboxylic acids with isobutene is a gentle method to form tert‑butyl esters. Here isobutene and the carboxylic acid react in the presence of a strong acid like sulfuric acid.
- The reaction of carboxylic acids with diazoalkanes is a very gentle and quantitative method to form esters. It is used primarily for the formation of methyl and benzyl esters on account of the inaccesibility of compex diazoalkanes.
Besides these classical methods for esterification, other modern techniques have been developed for special reactions.
- The activation of carboxylic acids with dicyclohexylcarbodiimide and reaction of the so-formed O‑acylisoureas with the alcoholic compound in the presence of 4‑N,N‑dimethylaminopyridine (the Steglich esterification).
- Activation of a carboxylic acid with formation of a mixed anhydride with 2,4,6-trichlorobenzoic acid via reaction of the carboxylic acid with benzoyl chloride in the presence of 4‑N,N‑dimethylaminopyridine and triethylamine. The mixed anhydride is formed in situ and immediately reacted with the alcoholic compound (Yamaguchi esterification).
- Activation of the alcoholic compound via reaction under Mitsunobu conditions with diethylazodicarboxylate and triphenylphosphine and associated reaction in situ with the carboxylic acid (Mitsunobu esterification).
Many groups can suffice for the alcoholic component. Here the methyl, tert-butyl, benzyl, and allyl esters are very commonly used. Moreover, a whole family of protecting groups are formed therefrom, which derive from the ester protection of the hydroxy group. The specific cleaving conditions are contrariwise generally quite similar. Basically, each ester can be hydrolyzed in the presence of hydroxide ions in a mixed water-alcohol solution. For sensitive substrates, it is typically appropriate to apply lithium hydroxide in tetrahydrofuran and methanol. From the hydrolytic tendencies the same rules naturally apply to esters as a protecting group for alcohols.
Name | Formula | Abbrev. | Cleavage | Special formation [sic?] |
---|---|---|---|---|
Methyl | ![]() |
Me | Nucleophilic/alkali with a metal hydroxide or carbonate in wet methanol or tetrahydrofuran,[71][72] alkali-halogen salts in wet aprotic solutions like dimethyl sulfoxide or heated N,N‑dimethylformamide,[73][74][75] or enzymatic, i. e. with pig liver esterase[76][77] | Diazomethane in diethyl ether,[78][79][80] caesium carbonate and methyl iodide in N,N‑Dimethylformamide,[81] or methanol and catalytic trimethylsilyl chloride[82] |
tert‑Butyl | ![]() |
tert‑Bu | acid: trifluoroacetic acid (pure or in dichloromethane),[83] formic acid, or p‑toluenesulfonic acid[84] | Isobutene in dioxane and catalytic sulfuric acid[85][86][87] |
Benzyl | ![]() |
Bn | Hydrogenolytic: hydrogen/palladium on activated carbon[88] | |
Benzhydryl | ![]() |
hydrogenolytic: hydrogen/palladium on activated carbon (very easy to cleave)[89] | ||
Allyl | ![]() |
Allyl | Similar to ethers: potassium tert‑butoxide, palladium on activated carbon, DABCO (1,4‑diazabicyclo[2.2.2]octane) in methanol, diverse platinum complexes – connected with acid workup[90] |
Protection of carboxylic acids:
- Methyl esters – Removed by acid or base.
- Benzyl esters – Removed by hydrogenolysis.
- tert-Butyl esters – Removed by acid, base and some reductants.
- Esters of 2,6-disubstituted phenols (e.g. 2,6-dimethylphenol, 2,6-diisopropylphenol, 2,6-di-tert-butylphenol) – Removed at room temperature by DBU-catalyzed methanolysis under high-pressure conditions.[91]
- Silyl esters – Removed by acid, base and organometallic reagents.
- Orthoesters – Removed by mild aqueous acid to form ester, which is removed according to ester properties.
- Oxazoline – Removed by strong hot acid (pH < 1, T > 100 °C) or alkali (pH > 12, T > 100 °C), but not e.g. LiAlH4, organolithium reagents or Grignard (organomagnesium) reagents
Phosphate protecting groups
- 2-cyanoethyl – removed by mild base. The group is widely used in oligonucleotide synthesis.
- Methyl (Me) – removed by strong nucleophiles e.c. thiophenole/TEA.
Terminal alkyne protecting groups
- Propargyl alcohols in the Favorskii reaction,
- Silyl groups, especially in protection of the acetylene itself.[92]
Other
- Photolabile protecting groups As a proof of concept orthogonal deprotection is demonstrated in a photochemical transesterification by trimethylsilyldiazomethane utilizing the kinetic isotope effect:[93]
Due to this effect the quantum yield for deprotection of the right-side ester group is reduced and it stays intact. Significantly by placing the deuterium atoms next to the left-side ester group or by changing the wavelength to 254 nm the other monoarene is obtained.
Criticism
The use of protective groups is pervasive but not without criticism.[94] In practical terms their use adds two steps (protection-deprotection sequence) to a synthesis, either or both of which can dramatically lower chemical yield. Crucially, added complexity impedes the use of synthetic total synthesis in drug discovery. In contrast biomimetic synthesis does not employ protective groups. As an alternative, Baran presented a novel protective-group free synthesis of the compound hapalindole U. The previously published synthesis[95][96][97] according to Baran, contained 20 steps with multiple protective group manipulations (two confirmed):
![]() |
![]() |
Industrial applications
Although the use of protecting groups is not preferred in industrial syntheses, they are still used in industrial contexts, e.g.:
- Oseltamivir (Tamiflu, an antiviral drug) Roche synthesis
- Sucralose (sweetener)
References
- ^ Theodora W. Greene; Peter G. M. Wuts (1999). Protecting Groups in Organic Synthesis (3 ed.). J. Wiley. ISBN 978-0-471-16019-9.
- ^ Chan, Weng C.; White, Peter D. (2004). Fmoc Solid Phase Peptide Synthesis. Oxford University Press. ISBN 978-0-19-963724-9.
- ^ Weng C. Chan, Peter D. White: Fmoc Solid Phase Peptide Synthesis, S. 10–12.
- ^ Merrifield, R. B.; Barany, G.; Cosand, W. L.; Engelhard, M.; Mojsov, S. (1977). "Proceedings of the 5th American Peptide Symposium". Biochemical Education. 7 (4): 93–94. doi:10.1016/0307-4412(79)90078-5.
- ^ Michael Schelhaas, Herbert Waldmann: „Schutzgruppenstrategien in der organischen Synthese“, in: Angewandte Chemie, 1996, 103, pp. 2195–2200; doi:10.1002/ange.19961081805 (in German).
- ^ V.N. Rajasekharan Pillai: „Photoremovable Protecting Groups in Organic Synthesis“, in: Synthesis, 1980, pp. 1–26.
- ^ P.J. Kocieński: Protecting Groups, p. 29.
- ^ P.J. Kocieński: Protecting Groups, p. 31.
- ^ Tod K Jones, Robert A. Reamer, Richard Desmond, Sander G. Mills: „Chemistry of tricarbonyl hemiketals and application of Evans technology to the total synthesis of the immunosuppressant (−)-FK-506“, in: J. Am. Chem. Soc., 1990, 112, pp. 2998–3017; doi:10.1021/ja00164a023.
- ^ Dieter Seebach, Hak-Fun Chow, Richard F.W. Jackson, Marius A. Sutter, Suvit Thaisrivongs, Jürg Zimmermann: „(+)-11,11′-Di-O-methylelaiophylidene – preparation from elaiophylin and total synthesis from (R)-3-hydroxybutyrate and (S)-malate“, in: Liebigs Ann. Chem., 1986, pp. 1281–1308; doi:10.1002/jlac.198619860714.
- ^ David A. Evans, Stephen W. Kaldor, Todd K. Jones, Jon Clardy, Thomas J. Stout: „Total synthesis of the macrolide antibiotic cytovaricin“, in: J. Am. Chem. Soc., 1990, 112, pp. 7001–7031; doi:10.1021/ja00175a038.
- ^ James A. Marshall, Richard Sedrani: „A convergent, highly stereoselective synthesis of a C-11-C-21 subunit of the macbecins“, in: J. Org. Chem., 1991, 56, pp. 5496–5498; doi:10.1021/jo00019a004.
- ^ James D. White, Motoji Kawasaki: „Total synthesis of (+)-latrunculin A“, in: J. Am. Chem. Soc., 1990, 112, pp. 4991–4993; doi:10.1021/ja00168a071.
- ^ Morris J. Robins, Vicente Samano, Mark D. Johnson: „Nucleic acid-related compounds. 58. Periodinane oxidation, selective primary deprotection, and remarkably stereoselective reduction of tert-butyldimethylsilyl-protected ribonucleosides. Synthesis of 9-(β-D-xylofuranosyl)adenine or 3'-deuterioadenosine from adenosine“, in: J. Org. Chem., 1990, 55, pp. 410–412; doi:10.1021/jo00289a004.
- ^ R. Roger F. Newton, Derek P. Reynolds, Colin F. Webb, Stanley M. Roberts: „A short and efficient total synthesis of (±) prostaglandin D2 methyl ester involving a new method for the cleavage of a dimethyl-t-butylsilyl ether“, in: J. Chem. Soc., Perkin Trans. 1, 1981, pp. 2055–2058; doi:10.1039/P19810002055.
- ^ Kyriacos C. Nicolaou, R. A. Daines, T. K. Chakraborty: „Total synthesis of amphoteronolide B“, in: J. Am. Chem. Soc., 1987, 109, pp. 2208–2210; doi:10.1021/ja00241a063.
- ^ Leo A. Paquette, Annette M. Doherty, Christopher M. Rayner: „Total synthesis of furanocembranolides. 1. Stereocontrolled preparation of key heterocyclic building blocks and assembly of a complete seco-pseudopterane framework“, in: J. Am. Chem. Soc., 1991, 109, pp. 3910–3926; doi:10.1021/ja00036a045.
- ^ P.J. Kocieński: Protecting Groups, p. 40.
- ^ P.J. Kocieński: Protecting Groups, pp. 46–49.
- ^ Michel Bessodes, Dimitri Komiotis, Kostas Antonakis: „Rapid and selective detritylation of primary alcohols using formic acid“, in: Tetrahedron Lett., 1986, 27, pp. 579–580; doi:10.1016/S0040-4039(00)84045-9.
- ^ B. Helferich: Carbonhydr. Chem. Biochem., 1948, 3, pp. 79.
- ^ M.L. García, J. Pascual, L. Borràs, J.A. Andreu, E. Fos, D. Mauleón, G. Carganico, F. Arcamone: „Synthesis of new ether glycerophospholipids structurally related to modulator“, in: Tetrahedron, 1991, 47, pp. 10023–10034; doi:10.1016/S0040-4020(01)96051-X.
- ^ Yuji Oikawa, Tadao Yoshioka, Osamu Yonemitsu: „Specific removal of o-methoxybenzyl protection by DDQ oxidation“, in: Tetrahedron Lett., 1982, 23, pp. 885–888; doi:10.1016/S0040-4039(00)86974-9.
- ^ See literature for p‑methoxybenzyl.
- ^ P.J. Kocieński: Protecting Groups, p. 77.
- ^ H. Nagaoka, W. Rutsch, G. Schmidt, H. Ito, M.R. Johnson, Y. Kishi: „Total synthesis of rifamycins. 1. Stereocontrolled synthesis of the aliphatic building block“, in: J. Am. Chem. Soc., 1980, 102, pp. 7962–7965; doi:10.1021/ja00547a037.
- ^ W. Clark Still, Dominick Mobilio: „Synthesis of asperdiol“, in: J. Org. Chem., 1983, 48, pp. 4785–4786; doi:10.1021/jo00172a070.
- ^ Masahiro Hirama, Mitsuko Uei: „A chiral total synthesis of compactin“, in: J. Am. Chem. Soc., 1982, 104, pp. 4251–4253; doi:10.1021/ja00379a037.
- ^ W. Clark Still, Shizuaki Murata, Gilbert Revial, Kazuo Yoshihara: „Synthesis of the cytotoxic germacranolide eucannabinolide“, in: J. Am. Chem. Soc., 1983, 105, pp. 625–627; doi:10.1021/ja00341a055.
- ^ Kamaya, Yasushi; T Higuchi (2006). "Metabolism of 3,4-dimethoxycinnamyl alcohol and derivatives by Coriolus versicolor". FEMS Microbiology Letters. 24 (2–3): 225–229. doi:10.1111/j.1574-6968.1984.tb01309.x.
- ^ Serge David, Annie Thieffry, Alain Veyrières: „A mild procedure for the regiospecific benzylation and allylation of polyhydroxy-compounds via their stannylene derivatives in non-polar solvents“, in: J. Chem. Soc., Perkin Trans. 1, 1981, pp. 1796–1801; doi:10.1039/P19810001796.
- ^ Kaoru Fuji, Shigetoshi Nakano, Eiichi Fujita: „An Improved Method for Methoxymethylation of Alcohols under Mild Acidic Conditions“, in: Synthesis, 1975, pp. 276–277.
- ^ Paul A. Wender, Carlos R. D. Correia: „Intramolecular photoinduced diene-diene cyaloadditions: a selective method for the synthesis of complex eight-membered rings and polyquinanes“, in: J. Am. Chem. Soc., 1987, 109, pp. 2523–2525; doi:10.1021/ja00242a053.
- ^ Karel F. Bernady, M. Brawner Floyd, John F. Poletto, Martin J. Weiss: „Prostaglandins and congeners. 20. Synthesis of prostaglandins via conjugate addition of lithium trans-1-alkenyltrialkylalanate reagents. A novel reagent for conjugate 1,4-additions“, in: J. Org. Chem., 1979, 44, pp. 1438–1447; doi:10.1021/jo01323a017.
- ^ Elias J. Corey, Haruki Niwa, Jochen Knolle: „Total synthesis of (S)-12-hydroxy-5,8,14-cis,-10-trans-eicosatetraenoic acid (Samuelsson's HETE)“, in: J. Am. Chem. Soc., 1978, 100, pp. 1942–1943; doi:10.1021/ja00474a058.
- ^ Elias J. Corey, Mark G. Bock: „Protection of primary hydroxyl groups as methylthiomethyl ethers“, in: Tetrahedron Lett., 1975, 16, pp. 3269–3270; doi:10.1016/S0040-4039(00)91422-9.
- ^ Elias J. Corey, Duy H. Hua, Bai Chuan Pan, Steven P. Seitz: „Total synthesis of aplasmomycin“, in: J. Am. Chem. Soc., 1982, 104, pp. 6818–6820; doi:10.1021/ja00388a074.
- ^ Robert C. Gadwood, Renee M. Lett, Jane E. Wissinger: „Total synthesis of (±)-poitediol and (±)4-epipoitediol“, in: J. Am. Chem. Soc., 1984, 106, pp. 3869–3870; doi:10.1021/ja00325a032.
- ^ James D. White, Motoji Kawasaki: „Total synthesis of (+)-latrunculin A“, in: J. Am. Chem. Soc., 1990, 112, pp. 4991–4993; doi:10.1021/ja00168a071.
- ^ Steven D. Burke, Gregory J. Pacofsky: „The ester enolate claisen rearrangement. Total synthesis of (±)-ethisolide“, in: Tetrahedron Lett., 1986, 27, pp. 445–448; doi:10.1016/S0040-4039(00)85501-X.
- ^ Toshiyuki Kan, Masaru Hashimoto, Mitsutoshi Yanagiya, Haruhisa Shirahama: „Effective deprotection of 2-(trimethylsilylethoxy)methylated alcohols (SEM ethers). Synthesis of thyrsiferyl-23 acetate“, in: Tetrahedron Lett., 1988, 29, pp. 5417–5418; doi:10.1016/S0040-4039(00)82883-X.
- ^ Joseph P. Marino, Scott L. Dax: „An efficient desilylation method for the generation of o-quinone methides: application to the synthesis of (+)- and (−)-hexahydrocannabinol“, in: J. Org. Chem., 1984, 49, pp. 3671–3672; doi:10.1021/jo00193a051.
- ^ P.J. Kocieński: Protecting Groups, pp. 59–60.
- ^ P.J. Kocieński: Protecting Groups, p. 62.
- ^ R.E. Ireland, D.W. Norbeck: „Convergent synthesis of polyether ionophore antibiotics: the synthesis of the monensin bis(tetrahydrofuran) via the Claisen rearrangement of an ester enolate with a β-leaving group“, in: J. Am. Chem. Soc., 1985, 107, pp. 3279–3285; doi:10.1021/ja00297a038.
- ^ András Lipták, János Imre, János Harangi, Pál Nánási, András Neszmélyi: „Chemo-, stereo- and regioselective hydrogenolysis of carbohydrate benzylidene acetals. Synthesis of benzyl ethers of benzyl α-D-, methyl β-D-mannopyranosides and benzyl α-D-rhamnopyranoside by ring cleavage of benzylidene derivatives with the LiAlH4-AlCl3 reagent“, in: Tetrahedron, 1982, 38, pp. 3721–3727; doi:10.1016/0040-4020(82)80083-5.
- ^ James A. Marshall, Joseph D. Trometer, Bruce E. Blough, Thomas D. Crute: „Stereochemistry of SN2' additions to acyclic vinyloxiranes“, in J. Org. Chem., 1988, 53, pp. 4274–4282 doi:10.1021/jo00253a020.
- ^ P.J. Kocieński: Protecting Groups, p. 186.
- ^ John O. Osby, Michael G. Martin, Bruce Ganem: An Exceptionally Mild Deprotection of Phthalimides, in: Tetrahedron Lett., 1984, 25, pp. 2093–2096; doi:10.1016/S0040-4039(01)81169-2.
- ^ P.J. Kocieński: Protecting Groups, pp. 220–227.
- ^ P.J. Kocieński: Protecting Groups, p. 195.
- ^ Robert M. Williams, Peter J. Sinclair, Dongguan Zhai, Daimo Chen: „Practical asymmetric syntheses of α-amino acids through carbon-carbon bond constructions on electrophilic glycine templates“, in: J. Am. Chem. Soc., 1988, 110, p. 1547–1557; doi:10.1021/ja00213a031.
- ^ Glenn L. Stahl, Roderich Walter, Clarck W. Smith: „General procedure for the synthesis of mono-N-acylated 1,6-diaminohexanes“, in: J. Org. Chem., 1978, 43, pp. 2285–2286; doi:10.1021/jo00405a045.
- ^ Naomi Sakai, Yasufumi Ohfune: „Total synthesis of galantin I. Acid-catalyzed cyclization of galantinic acid“, in: J. Am. Chem. Soc., 1992, 114, pp. 998–1010; doi:10.1021/ja00029a031.
- ^ Weng C. Chan, Peter D. White: Fmoc Solid Phase Peptide Synthesis, pp. 27–30.
- ^ Gregg B. Fields: Methods for Removing the Fmoc Group. (PDF; 663 kB) In: Michael W. Pennington, Ben M. Dunn (eds.): Peptide Synthesis Protocols volume 35, 1995, ISBN 978-0-89603-273-6, pp. 17–27.
- ^ B. Liebe, H. Kunz: Festphasensynthese eines tumorassoziierten Sialyl-Tn-Antigen-Glycopeptids mit einer Partialsequenz aus dem “Tandem Repeat” des MUC-1-Mucins In: Angew. Chem. volume 109, 1997, pp. 629–631 (in German).
- ^ ChemPep Inc.: Fmoc Solid Phase Peptide Synthesis. retrieved 16 November 2013.
- ^ P.J. Kocieński: Protecting Groups, pp. 199–201.
- ^ Moussa, Ziad; D. Romo (2006). "Mild deprotection of primary N-(p-toluenesufonyl) amides with SmI2 following trifluoroacetylation". Synlett. 2006 (19): 3294–3298. doi:10.1055/s-2006-951530.
- ^ T. Tsunoda, M. Suzuki, R. Noyori: „A facile procedure for acetalization under aprotic conditions“, in: Tetrahedron Lett., 1980, 21, pp. 1357–1358; doi:10.1016/S0040-4039(00)74575-8.
- ^ Juji Yoshimura, Shigeomi Horito, Hiroriobu Hashimoto: „Facile Synthesis of 2,3,4,6-Tetra-O-benzyl-D-glucopyranosylidene Acetals Using Trimethylsilyl Trifluoromethanesulfonate Catalyst“, in: Chem. Lett., 1981, 10, pp. 375–376; doi:10.1246/cl.1981.375.
- ^ Bruce H. Lipshutz, Daniel Pollart, Joseph Monforte, Hiyoshizo Kotsuki: „Pd(II)-catalyzed acetal/ketal hydrolysis/exchange reactions“, in: Tetrahedron Lett., 1985, 26, pp. 705–708; doi:10.1016/S0040-4039(00)89114-5.
- ^ Kwan Soo Kim, Yang Heon Song, Bong Ho Lee, Chi Sun Hahn: „Efficient and selective cleavage of acetals and ketals using ferric chloride adsorbed on silica gel“, in: J. Org. Chem., 1986, 51, pp. 404–407; doi:10.1021/jo00353a027.
- ^ P.J. Kocieński: Protecting Groups, S. 167–170.
- ^ P.J. Kocieński: Protecting Groups, pp. 176.
- ^ P.J. Kocieński: Protecting Groups, pp. 178–180.
- ^ Samuel J. Danishefsky, Nathan B. Mantlo, Dennis S. Yamashita, Gayle. Schulte: „Concise route to the calichemicin-esperamicin series: the crystal structure of an aglycone prototype“, in: J. Am. Chem. Soc., 1988, 110, pp. 6890–6891; doi:10.1021/ja00228a051.
- ^ John N. Haseltine, Maria Paz Cabal, Nathan B. Mantlo, Nobuharu Iwasawa, Dennis S. Yamashita, Robert S. Coleman, Samuel J. Danishefsky, Gayle K. Schulte: „Total synthesis of calicheamicinone: new arrangements for actuation of the reductive cycloaromatization of aglycon congeners“, in: J. Am. Chem. Soc., 1991, 113, pp. 3850–3866; doi:10.1021/ja00010a030.
- ^ P.J. Kocieński: Protecting Groups, pp. 119.
- ^ Satomi Niwayama: „Highly Efficient Selective Monohydrolysis of Symmetric Diesters“, in: J. Org. Chem., 2000, 65, pp. 5834–5836; doi:10.1021/jo0001986.
- ^ J.M. Khurana, Arti Sehgal: „An efficent and convenient procedure for ester hydrolysis“, in: Org. Prep. Proced. Ind., 1994, 26, pp. 580–583.
- ^ Robert V. Stevens, Albert W. M. Lee: „Stereochemistry of the Robinson-Schoepf reaction. A stereospecific total synthesis of the ladybug defense alkaloids precoccinelline and coccinelline“, in: J. Am. Chem. Soc., 1979, 101, pp. 7032–7035; doi:10.1021/ja00517a042.
- ^ J. Wrobel, K. Takahashi, V. Honkan, G. Lannoye, J. M. Cook, Steven H. Bertz: „α-Lithio ketones. 1. Stereocontrolled synthesis of (±)-modhephene via the Weiss reaction“, in: J. Org. Chem., 1983, 48, pp. 139–141; doi:10.1021/jo00149a034.
- ^ Dennis D. Keith, John A. Tortora, Roxana Yang: „Synthesis of L-2-amino-4-methoxy-trans-but-3-enoic acid“, in: J. Org. Chem., 1978, 43, pp. 3711–3713; doi:10.1021/jo00413a016.
- ^ Peter Mohr, Nada Waespe-Šarčević, Christoph Tamm, Krystyna Gawronska, Jacek K. Gawronski: „A Study of Stereoselective Hydrolysis of Symmetrical Diesters with Pig Liver Esterase“, in: Helv. Chim. Acta, 1983, 66, pp. 2501–2511; doi:10.1002/hlca.19830660815.
- ^ Théophile Tschamber, Nada Waespe-Šarčević, Christoph Tamm: „Stereocontrolled Synthesis of an Epimer of the C(19)-to-C(27) Segment of Rifamycin S“, in: Helv. Chim. Acta, 1986, 69, pp. 621–625; doi:10.1002/hlca.19860690311.
- ^ Yves Rubin, Carolyn B. Knobler, Francois Diederich: „Precursors to the cyclo[n]carbons: from 3,4-dialkynyl-3-cyclobutene-1,2-diones and 3,4-dialkynyl-3-cyclobutene-1,2-diols to cyclobutenodehydroannulenes and higher oxides of carbon“, in: J. Am. Chem. Soc., 1990, 112, pp. 1607–1617; doi:10.1021/ja00160a047.
- ^ Sunggak Kim, Yong Gil Kim, Deog-il Kim: „A novel method for selective dioxolanation of ketones in the presence of aldehydes“, in: Tetrahedron Lett., 1992, 33, pp. 2565–2566; doi:10.1016/S0040-4039(00)92243-3.
- ^ G. Bauduin, D. Bondon, Y. Pietrasanta, B. Pucci: „Reactions de transcetalisation – II: Influence des facteurs steriques et electroniques sur les energies de cetalisation“, in: Tetrahedron, 1978, 34, pp. 3269–3274; doi:10.1016/0040-4020(78)80243-9.
- ^ John E. McMurry, Stephen J. Isser: „Total synthesis of longifolene“, in: J. Am. Chem. Soc., 1972, 94, pp. 7132–7137; doi:10.1021/ja00775a044.
- ^ M.P. Bosch, M. Pilar Bosch, Francisco Camps, Jose Coll, Angel Guerrero, Toshio Tatsuoka, Jerrold Meinwald: „A stereoselective total synthesis of (±)-muzigadial“, in: J. Org. Chem., 1986, 51, pp. 773–784; doi:10.1021/jo00356a002.
- ^ Ulrich Schmidt, Thomas Beuttler, Albrecht Lieberknecht, Helmut Griesser: „Aminosäuren und peptide – XXXXII. Synthese von Chlamydocin + epi-Chlamydocin“, in: Tetrahedron Lett., 1983, 24, pp. 3573–3576; doi:10.1016/S0040-4039(00)88171-X.
- ^ Elias J. Corey, Plato A. Magriotis: „Total synthesis and absolute configuration of 7,20-diisocyanoadociane“, in: J. Am. Chem. Soc., 1987, 109, pp. 287–289; doi:10.1021/ja00235a052.
- ^ Elias J. Corey, Kyriacos C. Nicolaou, Takeshi Toru: „Total synthesis of (±)-vermiculine“, in: J. Am. Chem. Soc., 1975, 97, pp. 2287–2288; doi:10.1021/ja00841a058.
- ^ Tainejiro Hiyama, Akihiro Kanakura, Hajime Yamamoto, Hitosi Nozaki: „General Route to α,β-unsaturated Aldehydes of Homoterpenoid and terpenoid Structure. Sythesis of JH-II and β-Sinensal“, in: Tetrahedron Lett., 1978, 19, pp. 3051–3054; doi:10.1016/S0040-4039(01)94936-6.
- ^ F. Huet, A. Lechevallier, M. Pellet, J.M. Conia: „Wet Silica Gel; A Convenient Reagent for Deacetalization“, in: Synthesis, 1978, pp. 63–64.
- ^ F. Zymalkokowski: Katalytische Hydrierung, Ferdinand Enke Verlag, Stuttgart 1965, pp. 127–133.
- ^ P.J. Kocieński: Protecting Groups, pp. 136.
- ^ P.J. Kocieński: Protecting Groups, pp. 139–142.
- ^ Romanski, J.; Nowak, P.; Kosinski, K.; Jurczak, J. (September 2012). "High-pressure transesterification of sterically hindered esters". Tetrahedron Lett. 53 (39): 5287–5289. doi:10.1016/j.tetlet.2012.07.094.
- ^ Clayden, Jonathan; Greeves, Nick; Warren, Stuart; Wothers, Peter (2000). Organic Chemistry. Oxford University Press. pp. 1291. ISBN 978-0-19-850346-0.
- ^ Blanc, Aurélien; Bochet, Christian G. (2007). "Isotope Effects in Photochemistry: Application to Chromatic Orthogonality" (PDF). Org. Lett. 9 (14): 2649–2651. doi:10.1021/ol070820h. PMID 17555322.
- ^ Baran, Phil S.; Maimone, Thomas J.; Richter, Jeremy M. (22 March 2007). "Total synthesis of marine natural products without using protecting groups". Nature. 446 (7134): 404–408. Bibcode:2007Natur.446..404B. doi:10.1038/nature05569. PMID 17377577. S2CID 4357378.
- ^ Synthetic studies of marine alkaloids hapalindoles. Part I Total synthesis of (±)-hapalindoles J and M Tetrahedron, Volume 46, Issue 18, 1990, Pages 6331–6342 Hideaki Muratake and Mitsutaka Natsume doi:10.1016/S0040-4020(01)96005-3
- ^ Synthetic studies of marine alkaloids hapalindoles. Part 2. Lithium aluminum hydride reduction of the electron-rich carbon-carbon double bond conjugated with the indole nucleus Tetrahedron, Volume 46, Issue 18, 1990, Pages 6343–6350 Hideaki Muratake and Mitsutaka Natsume doi:10.1016/S0040-4020(01)96006-5
- ^ Synthetic studies of marine alkaloids hapalindoles. Part 3 Total synthesis of (±)-hapalindoles H and U Tetrahedron, Volume 46, Issue 18, 1990, Pages 6351–6360 Hideaki Muratake, Harumi Kumagami and Mitsutaka Natsume doi:10.1016/S0040-4020(01)96007-7
External links
- Introduction of protecting group and mechanism of deprotection
- Senior undergraduate study notes on this subject, from Prof. Rizzo.
- A further set of study notes in tutorial form, with guidance and comments, from Profs. Grossman and Cammers.
- A review by Prof. Kocienski.
- A user site excerpting the classic Greene and Wuts text regarding stability of a few key groups, from this reference's extensive tables.