Nickel aluminide: Difference between revisions
m link thermal conductivity using Find link |
HighTempMSE (talk | contribs) I effectively re-wrote the introduction section and added two sections specifically on Ni3Al and NiAl |
||
Line 1: | Line 1: | ||
'''Nickel aluminide''' typically refers to the one of the two most widely used compounds, Ni<sub>3</sub>Al or NiAl, however is generally any aluminide from the Ni-Al system. These alloys are widely used due to their corrosion resistance, low-density and easy production.<ref name=":0">{{Citation|last=Kurbatkina|first=Victoria V.|title=Nickel Aluminides|date=2017-01-01|url=https://www.sciencedirect.com/science/article/pii/B9780128041734000995|work=Concise Encyclopedia of Self-Propagating High-Temperature Synthesis|pages=212–213|editor-last=Borovinskaya|editor-first=Inna P.|place=Amsterdam|publisher=Elsevier|language=en|isbn=978-0-12-804173-4|access-date=2021-03-07|editor2-last=Gromov|editor2-first=Alexander A.|editor3-last=Levashov|editor3-first=Evgeny A.|editor4-last=Maksimov|editor4-first=Yuri M.}}</ref> Ni<sub>3</sub>Al is of specific interest as the strengthening γ' phase precipitate in nickel-based superalloys allowing for high temperature strength up to 0.7-0.8 of its melting temperature.<ref name=":0" /><ref name=":1">{{Cite journal|last=Dey|first=G. K.|date=2003-02-01|title=Physical metallurgy of nickel aluminides|url=https://doi.org/10.1007/BF02717135|journal=Sadhana|language=en|volume=28|issue=1|pages=247–262|doi=10.1007/BF02717135|issn=0973-7677}}</ref> Meanwhile, NiAl displays excellent properties such as low-density (lower than that of Ni<sub>3</sub>Al), good thermal conductivity, oxidation resistance and high melting temperature.<ref name=":1" /> These properties, make it ideal for special high temperature applications like coatings on blades in [[gas turbine]]s and [[jet engine]]s. However, both these alloys do have the disadvantage of being quite brittle at room temperature while Ni<sub>3</sub>Al remains brittle at high temperatures as well.<ref name=":0" /> Although, it has been shown that Ni<sub>3</sub>Al can be made ductile when manufactured as a single crystal as opposed to polycrystalline.<ref>{{Cite journal|last=Pope|first=D. P.|last2=Ezz|first2=S. S.|date=1984-01-01|title=Mechanical properties of Ni3AI and nickel-base alloys with high volume fraction of γ'|url=https://doi.org/10.1179/imtr.1984.29.1.136|journal=International Metals Reviews|volume=29|issue=1|pages=136–167|doi=10.1179/imtr.1984.29.1.136|issn=0308-4590}}</ref> Another application was demonstrated in 2005, when the most abrasion-resistant material was reportedly created by embedding [[diamond]]s in a matrix of nickel aluminide.<ref>[http://www.azom.com/news.asp?newsID=3866 Scientists Develop Nickel Aluminide Composite Material that Can Cut Through Cast Iron and Granite]</ref> |
|||
'''Nickel aluminide''' (Ni<sub>3</sub>Al) is an [[intermetallic alloy]] of [[nickel]] and [[aluminium]] with properties similar to both a ceramic and a metal. |
|||
⚫ | |||
There are three materials called nickel aluminide: |
|||
The predominate issue with polycrystalline Ni<sub>3</sub>Al-based alloys is the room temperature and high temperature brittleness. This brittleness is generally attributed to the inability for dislocations to move in the highly ordered lattices.<ref>{{Cite journal|last=Wu|first=Yu-ting|last2=Li|first2=Chong|last3=Li|first3=Ye-fan|last4=Wu|first4=Jing|last5=Xia|first5=Xing-chuan|last6=Liu|first6=Yong-chang|date=2020|title=Effects of heat treatment on the microstructure and mechanical properties of Ni3Al-based superalloys: A review|url=http://ijmmm.ustb.edu.cn/en/article/doi/10.1007/s12613-020-2177-y|journal=International Journal of Minerals, Metallurgy and Materials|doi=10.1007/s12613-020-2177-y|issn=1674-4799}}</ref> Researchers worked hard to address this brittleness as it greatly reduced the potential structural applications these Ni<sub>3</sub>Al-based alloys could be used for. However, in 1990, it was shown that the introduction of small amount of boron can drastically increase the ductility by suppressing intergranular fracture.<ref>{{Cite journal|last=K|first=Aoki|date=1990|title=Ductilization of L12 Intermetallic Compound Ni3Al by Microalloying with Boron|url=https://www.jstage.jst.go.jp/article/matertrans1989/31/6/31_6_443/_article|journal=Materials Transactions, JIM|volume=31|issue=6|pages=443-448|doi=10.2320/matertrans1989.31.443|via=J-STAGE}}</ref> Once this was addressed focus turned to maximizing the structural properties of the alloy. As mentioned, NiAl<sub>3</sub>-based alloys derive their strength from the formation of γ' precipitates in the γ which strength the alloys through precipitate strengthening. In these NiAl<sub>3</sub>-based alloys the volume fraction of the γ' precipitates is as high as 80%.<ref name=":2">{{Cite journal|last=Wu|first=Yuting|last2=Liu|first2=Yongchang|last3=Li|first3=Chong|last4=Xia|first4=Xingchuan|last5=Wu|first5=Jing|last6=Li|first6=Huijun|date=2019-01-15|title=Coarsening behavior of γ′ precipitates in the γ'+γ area of a Ni3Al-based alloy|url=https://www.sciencedirect.com/science/article/pii/S0925838818331542|journal=Journal of Alloys and Compounds|language=en|volume=771|pages=526–533|doi=10.1016/j.jallcom.2018.08.265|issn=0925-8388}}</ref> Due to this high volume fraction the evolution of these γ' precipitates during the life cycles of these alloys has been a field of great interest. One of the main concerns is the coarsening of these γ' precipitates at high temperature (800°C to 1000°C) which greatly reduces the strength of these alloys.<ref name=":2" /> This coarsening is due to the balance between interfacial and elastic energy in the γ + γ' phase and is generally inevitable over long durations of time.<ref name=":2" /> Current research has attempted to address this coarsening issue by introducing other elements. Elements such as Fe, Cr and Mo have shown to create unique multiphase configurations that can significantly increase NiAl<sub>3</sub>-based alloys' creep resistance at 1000°C for 1000 hours.<ref name=":3">{{Cite journal|last=Wu|first=Jing|last2=Li|first2=Chong|last3=Wu|first3=Yuting|last4=Huang|first4=Yuan|last5=Xia|first5=Xingchuan|last6=Liu|first6=Yongchang|date=2020-07-14|title=Creep behaviors of multiphase Ni3Al-based intermetallic alloy after 1000°C-1000h long-term aging at intermediate temperatures|url=https://www.sciencedirect.com/science/article/pii/S0921509320307796|journal=Materials Science and Engineering: A|language=en|volume=790|pages=139701|doi=10.1016/j.msea.2020.139701|issn=0921-5093}}</ref> This creep resistance is attributed to the formation of inhomogeneous precipitate Cr<sub>4.6</sub>MoNi<sub>2.1</sub> which pins dislocations and prevents further coarsening of the γ' phase.<ref name=":3" /> This addition of Fe and Cr also drastically increases the weldability of the NiAl<sub>3</sub>-based alloy which still posed a significant issue for industrial use despite its easy and cost effective production.<ref name=":3" /> In general, Ni<sub>3</sub>Al acts as an excellent strengthening precipitate in Ni-based alloys making these materials ideal for high temperature, load bearing applications. Further research is being done to address the pitfalls of this material via the incorporation of other elements. |
|||
* NiAl, [[CAS number]] 12003-78-0 (see also [[Raney nickel]]) |
|||
* NiAl<sub>3</sub>, [[CAS number]] 12004-71-6 |
|||
⚫ | |||
== NiAl == |
|||
Nickel aluminide is used as a strengthening constituent in high-temperature nickel-base [[superalloy]]s, however, unalloyed nickel aluminide has a tendency to exhibit brittle fracture and low ductility at ambient temperatures.<ref>[http://www.asminternational.org/content/ASM/StoreFiles/ACFA9D7.pdf "ASM Specialty Handbook: Nickel, Cobalt, and Their Alloys", p. 104]</ref> |
|||
NiAl despite its beneficial properties generally suffers from two factors: very high brittleness at low temperatures (<330°C) and rapid loss of strength for temperatures higher than 550°C.<ref name=":4">{{Cite journal|last=Czeppe|first=Tomasz|last2=Wierzbinski|first2=Stanislaw|date=2000-08-01|title=Structure and mechanical properties of NiAl and Ni3Al-based alloys|url=https://www.sciencedirect.com/science/article/pii/S0020740399000879|journal=International Journal of Mechanical Sciences|language=en|volume=42|issue=8|pages=1499–1518|doi=10.1016/S0020-7403(99)00087-9|issn=0020-7403}}</ref> The brittleness is attributed to both the high energy of anti-phase boundaries as well as high atomic order along grain boundaries.<ref name=":4" /> Similar to that of Ni<sub>3</sub>Al-based alloys these issues are generally addressed via the integration of other elements. Attempted elements can be broken into three groups depending on their influence of microstructure: |
|||
Nickel aluminide is unique in that it has very high [[thermal conductivity]] combined with high strength at high temperature. These properties, combined with its high strength and low density, make it ideal for special applications like coating blades in [[gas turbine]]s and [[jet engine]]s. |
|||
* Elements that form ternary intermetallic phases such as Ti and Hf<ref name=":4" /> |
|||
In 2005, the most abrasion-resistant material was reportedly created by embedding [[diamond]]s in a matrix of nickel aluminide.<ref>[http://www.azom.com/news.asp?newsID=3866 Scientists Develop Nickel Aluminide Composite Material that Can Cut Through Cast Iron and Granite]</ref> |
|||
* Pseudobinary eutectic forming elements such as Cr<ref name=":4" /> |
|||
* Elements with high solubility in NiAl such as Fe, Co and Cu<ref name=":4" /> |
|||
Some of the more successful elements have been shown to be Fe, Co and Cr which drastically increase room temperature ductility as well as hot workability.<ref name=":5">{{Cite journal|last=Ishida|first=K.|last2=Kainuma|first2=R.|last3=Ueno|first3=N.|last4=Nishizawa|first4=T.|date=1991-02-01|title=Ductility enhancement in NiAl (B2)-base alloys by microstructural control|url=https://doi.org/10.1007/BF02656811|journal=Metallurgical Transactions A|language=en|volume=22|issue=2|pages=441–446|doi=10.1007/BF02656811|issn=1543-1940}}</ref> This increase is due to the formation of γ phase which modifies the β phase grains.<ref name=":5" /> Alloying with Fe, Ga and Mo has also been show to drastically improve room temperature ductility as well.<ref>{{Cite journal|last=Darolia|first=Ram|date=1991-03-01|title=NiAl alloys for high-temperature structural applications|url=https://doi.org/10.1007/BF03220163|journal=JOM|language=en|volume=43|issue=3|pages=44–49|doi=10.1007/BF03220163|issn=1543-1851}}</ref> Most recently, refracturing metals such as Cr, W and Mo have been added and resulted in not only increases in room temperature ductility but also increases in strength and fracture toughness at high temperatures.<ref name=":6">{{Cite journal|last=Khomutov|first=M.|last2=Potapkin|first2=P.|last3=Cheverikin|first3=V.|last4=Petrovskiy|first4=P.|last5=Travyanov|first5=A.|last6=Logachev|first6=I.|last7=Sova|first7=A.|last8=Smurov|first8=I.|date=2020-05-01|title=Effect of hot isostatic pressing on structure and properties of intermetallic NiAl–Cr–Mo alloy produced by selective laser melting|url=https://www.sciencedirect.com/science/article/pii/S0966979519310775|journal=Intermetallics|language=en|volume=120|pages=106766|doi=10.1016/j.intermet.2020.106766|issn=0966-9795}}</ref> This is due to the formation of unique microstructures such as the eutectic alloy Ni<sub>45.5</sub>Al<sub>9</sub>Mo and α-Cr inclusions that contribute to solid solution hardening.<ref name=":6" /> It is even being shown that these complex alloys (Ni<sub>42</sub>Al<sub>51</sub>Cr<sub>3</sub>Mo<sub>4</sub>) have the potential to be fabricated via additive manufacturing processes such as [[Selective laser melting|selective laser manufacturing]], vastly increasing the potential applications for these alloys.<ref name=":6" /> |
|||
==IC-221M== |
==IC-221M== |
Revision as of 02:46, 8 March 2021
Nickel aluminide typically refers to the one of the two most widely used compounds, Ni3Al or NiAl, however is generally any aluminide from the Ni-Al system. These alloys are widely used due to their corrosion resistance, low-density and easy production.[1] Ni3Al is of specific interest as the strengthening γ' phase precipitate in nickel-based superalloys allowing for high temperature strength up to 0.7-0.8 of its melting temperature.[1][2] Meanwhile, NiAl displays excellent properties such as low-density (lower than that of Ni3Al), good thermal conductivity, oxidation resistance and high melting temperature.[2] These properties, make it ideal for special high temperature applications like coatings on blades in gas turbines and jet engines. However, both these alloys do have the disadvantage of being quite brittle at room temperature while Ni3Al remains brittle at high temperatures as well.[1] Although, it has been shown that Ni3Al can be made ductile when manufactured as a single crystal as opposed to polycrystalline.[3] Another application was demonstrated in 2005, when the most abrasion-resistant material was reportedly created by embedding diamonds in a matrix of nickel aluminide.[4]
Ni3Al
The predominate issue with polycrystalline Ni3Al-based alloys is the room temperature and high temperature brittleness. This brittleness is generally attributed to the inability for dislocations to move in the highly ordered lattices.[5] Researchers worked hard to address this brittleness as it greatly reduced the potential structural applications these Ni3Al-based alloys could be used for. However, in 1990, it was shown that the introduction of small amount of boron can drastically increase the ductility by suppressing intergranular fracture.[6] Once this was addressed focus turned to maximizing the structural properties of the alloy. As mentioned, NiAl3-based alloys derive their strength from the formation of γ' precipitates in the γ which strength the alloys through precipitate strengthening. In these NiAl3-based alloys the volume fraction of the γ' precipitates is as high as 80%.[7] Due to this high volume fraction the evolution of these γ' precipitates during the life cycles of these alloys has been a field of great interest. One of the main concerns is the coarsening of these γ' precipitates at high temperature (800°C to 1000°C) which greatly reduces the strength of these alloys.[7] This coarsening is due to the balance between interfacial and elastic energy in the γ + γ' phase and is generally inevitable over long durations of time.[7] Current research has attempted to address this coarsening issue by introducing other elements. Elements such as Fe, Cr and Mo have shown to create unique multiphase configurations that can significantly increase NiAl3-based alloys' creep resistance at 1000°C for 1000 hours.[8] This creep resistance is attributed to the formation of inhomogeneous precipitate Cr4.6MoNi2.1 which pins dislocations and prevents further coarsening of the γ' phase.[8] This addition of Fe and Cr also drastically increases the weldability of the NiAl3-based alloy which still posed a significant issue for industrial use despite its easy and cost effective production.[8] In general, Ni3Al acts as an excellent strengthening precipitate in Ni-based alloys making these materials ideal for high temperature, load bearing applications. Further research is being done to address the pitfalls of this material via the incorporation of other elements.
NiAl
NiAl despite its beneficial properties generally suffers from two factors: very high brittleness at low temperatures (<330°C) and rapid loss of strength for temperatures higher than 550°C.[9] The brittleness is attributed to both the high energy of anti-phase boundaries as well as high atomic order along grain boundaries.[9] Similar to that of Ni3Al-based alloys these issues are generally addressed via the integration of other elements. Attempted elements can be broken into three groups depending on their influence of microstructure:
- Elements that form ternary intermetallic phases such as Ti and Hf[9]
- Pseudobinary eutectic forming elements such as Cr[9]
- Elements with high solubility in NiAl such as Fe, Co and Cu[9]
Some of the more successful elements have been shown to be Fe, Co and Cr which drastically increase room temperature ductility as well as hot workability.[10] This increase is due to the formation of γ phase which modifies the β phase grains.[10] Alloying with Fe, Ga and Mo has also been show to drastically improve room temperature ductility as well.[11] Most recently, refracturing metals such as Cr, W and Mo have been added and resulted in not only increases in room temperature ductility but also increases in strength and fracture toughness at high temperatures.[12] This is due to the formation of unique microstructures such as the eutectic alloy Ni45.5Al9Mo and α-Cr inclusions that contribute to solid solution hardening.[12] It is even being shown that these complex alloys (Ni42Al51Cr3Mo4) have the potential to be fabricated via additive manufacturing processes such as selective laser manufacturing, vastly increasing the potential applications for these alloys.[12]
IC-221M
An alloy of Ni3Al, known as IC-221M, is made up of nickel aluminide combined with several other metals including chromium, molybdenum, zirconium and boron. Adding boron increases the ductility of the alloy by positively altering the grain boundary chemistry and promoting grain refinement. The Hall-Petch parameters for this material were σo = 163 MPa and ky = 8.2 MPaˑcm1/2.[13] Boron increases the hardness of bulk Ni3Al by a similar mechanism.
This alloy is extremely strong for its weight, five times stronger than common SAE 304 stainless steel. Unlike most alloys, IC-221M increases in strength from room temperature up to 800 °C.
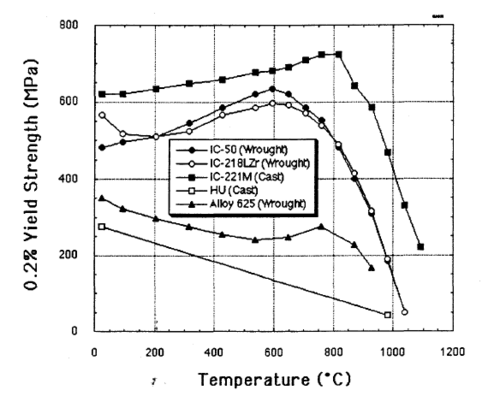
The alloy is very resistant to heat and corrosion, and finds use in heat-treating furnaces and other applications where its longer lifespan and reduced corrosion give it an advantage over stainless steel.[14]
Properties
- Ni3Al has a cubic crystalline structure of the L12 type, with lattice parameter a = 355.9 pm.
- Density = 7.16 g/cm3
- Yield Strength = 855 MPa
- Hardness = HRC 12
- Thermal Conductivity Ni3Al = 28.85 (W/m.K)[15]
- Thermal Conductivity NiAl = 76 (W/m.K) [15]
- Melting Point Ni3Al = 1668 K
- Melting Point NiAl = 1955 K
- Thermal expansion coefficient = 12.5 (10−6/K)
- Bonding = covalent/metallic
- Electrical resistivity = 32.59 (10−8Ωm)
References
- ^ a b c Kurbatkina, Victoria V. (2017-01-01), Borovinskaya, Inna P.; Gromov, Alexander A.; Levashov, Evgeny A.; Maksimov, Yuri M. (eds.), "Nickel Aluminides", Concise Encyclopedia of Self-Propagating High-Temperature Synthesis, Amsterdam: Elsevier, pp. 212–213, ISBN 978-0-12-804173-4, retrieved 2021-03-07
- ^ a b Dey, G. K. (2003-02-01). "Physical metallurgy of nickel aluminides". Sadhana. 28 (1): 247–262. doi:10.1007/BF02717135. ISSN 0973-7677.
- ^ Pope, D. P.; Ezz, S. S. (1984-01-01). "Mechanical properties of Ni3AI and nickel-base alloys with high volume fraction of γ'". International Metals Reviews. 29 (1): 136–167. doi:10.1179/imtr.1984.29.1.136. ISSN 0308-4590.
- ^ Scientists Develop Nickel Aluminide Composite Material that Can Cut Through Cast Iron and Granite
- ^ Wu, Yu-ting; Li, Chong; Li, Ye-fan; Wu, Jing; Xia, Xing-chuan; Liu, Yong-chang (2020). "Effects of heat treatment on the microstructure and mechanical properties of Ni3Al-based superalloys: A review". International Journal of Minerals, Metallurgy and Materials. doi:10.1007/s12613-020-2177-y. ISSN 1674-4799.
- ^ K, Aoki (1990). "Ductilization of L12 Intermetallic Compound Ni3Al by Microalloying with Boron". Materials Transactions, JIM. 31 (6): 443–448. doi:10.2320/matertrans1989.31.443 – via J-STAGE.
- ^ a b c Wu, Yuting; Liu, Yongchang; Li, Chong; Xia, Xingchuan; Wu, Jing; Li, Huijun (2019-01-15). "Coarsening behavior of γ′ precipitates in the γ'+γ area of a Ni3Al-based alloy". Journal of Alloys and Compounds. 771: 526–533. doi:10.1016/j.jallcom.2018.08.265. ISSN 0925-8388.
- ^ a b c Wu, Jing; Li, Chong; Wu, Yuting; Huang, Yuan; Xia, Xingchuan; Liu, Yongchang (2020-07-14). "Creep behaviors of multiphase Ni3Al-based intermetallic alloy after 1000°C-1000h long-term aging at intermediate temperatures". Materials Science and Engineering: A. 790: 139701. doi:10.1016/j.msea.2020.139701. ISSN 0921-5093.
- ^ a b c d e Czeppe, Tomasz; Wierzbinski, Stanislaw (2000-08-01). "Structure and mechanical properties of NiAl and Ni3Al-based alloys". International Journal of Mechanical Sciences. 42 (8): 1499–1518. doi:10.1016/S0020-7403(99)00087-9. ISSN 0020-7403.
- ^ a b Ishida, K.; Kainuma, R.; Ueno, N.; Nishizawa, T. (1991-02-01). "Ductility enhancement in NiAl (B2)-base alloys by microstructural control". Metallurgical Transactions A. 22 (2): 441–446. doi:10.1007/BF02656811. ISSN 1543-1940.
- ^ Darolia, Ram (1991-03-01). "NiAl alloys for high-temperature structural applications". JOM. 43 (3): 44–49. doi:10.1007/BF03220163. ISSN 1543-1851.
- ^ a b c Khomutov, M.; Potapkin, P.; Cheverikin, V.; Petrovskiy, P.; Travyanov, A.; Logachev, I.; Sova, A.; Smurov, I. (2020-05-01). "Effect of hot isostatic pressing on structure and properties of intermetallic NiAl–Cr–Mo alloy produced by selective laser melting". Intermetallics. 120: 106766. doi:10.1016/j.intermet.2020.106766. ISSN 0966-9795.
- ^ Liu, C. T.; White, C. L.; Horton, J. A. (1985). "Effect of boron on grain-boundaries in Ni3Al". Acta Metall. 33 (2): 213–229. doi:10.1016/0001-6160(85)90139-7.
- ^ Crawford, Gerald (April 2003). "Exotic Alloy Finds Niche". Nickel magazine. Retrieved 2006-12-19.
- ^ a b Dey, G. K. (2003). "Physical Metallurgy of Nickel Aluminides" (PDF). Sadhana. 28 (Parts 1 & 2): 247–262. doi:10.1007/bf02717135. Retrieved 2014-03-05.