Wind power: Difference between revisions
→Offshore: additions and style changes |
|||
Line 164: | Line 164: | ||
===Offshore=== |
===Offshore=== |
||
[[Image:DanishWindTurbines.jpg|thumb|500px|Wind |
[[Image:DanishWindTurbines.jpg|thumb|500px|Wind blows briskly and smoothly over water since there are no obstructions. The large and slow turning turbines of this offshore wind farm near Copenhagen take advantage of the moderate yet constant breezes here.]] |
||
Offshore wind turbines are considered to be less unsightly |
Offshore wind turbines are considered to be less unsightly since they can be invisible from shore. Because there are fewer obstacles and stronger winds, such turbines don´t need to be built as high into the air. However, offshore conditions are harsh, abrasive, and corrosive, and it is more difficult to maintain a turbine in open waters than on land. |
||
In |
In areas with extended shallow continental shelves and sand banks (such as [[Denmark]]), turbines are reasonably easy to install, and give good service. At the site shown, the wind is not especially strong but is very consistent. The largest offshore wind turbines in the world are seven 3.6 MW rated machines off the east coast of Ireland about 60 km south of Dublin. The turbines are located on a sandbank approximately 10 km from the coast that has the potential for the installation of 500 MW of generation capacity. |
||
[[As of 2006]], the largest offshore wind farm is Horns Rev which is located |
[[As of 2006]], the largest offshore wind farm is Horns Rev which is located 15 km west off the West coast of [[Jutland]], [[Denmark]] and produces 160 MW of power.<ref>{{cite web| url=http://www.hornsrev.dk/Engelsk/default_ie.htm| title=Horns Rev offshore wind farm| publisher=Elsam| accessdate=2006-04-21}}</ref> |
||
===Airborne=== |
===Airborne=== |
Revision as of 13:21, 26 May 2006
You must add a |reason=
parameter to this Cleanup template – replace it with {{Cleanup|May 22, 2006|reason=<Fill reason here>}}
, or remove the Cleanup template.
In 2005, worldwide capacity of wind-powered generators was 58,982 megawatts, their production making up less than 1% of world-wide electricity use. Although still a relatively minor source of electricity for most countries, wind power generation more than quadrupled between 1999 and 2005.
Most modern wind power is generated in the form of electricity by converting the rotation of turbine blades into electrical current by means of an electrical generator. In windmills (a much older technology) wind energy is used to turn mechanical machinery to do physical work, like crushing grain or pumping water.
Wind power is used in large scale wind farms for national electrical grids as well as in small individual turbines for providing electricity in isolated locations.
Wind energy is abundant, inexhaustible, widely distributed, clean, and mitigates the greenhouse effect.
Economics
In recent years, the cost of most wind-generated electric power has dropped substantially. Since 2004, according to some sources, the price in the United States is now lower than the cost of fuel-generated electric power, even without taking externalities into account.[1][2][3] Wind power is growing quickly, at about 38%,[4] up from 25% growth in 2002. In the United States, as of 2003, wind power was the fastest growing form of electricity generation on a percentage basis.[5] In 2005, wind energy cost one-fifth as much as it did in the late 1990s, and that downward trend is expected to continue as larger multi-megawatt turbines are mass-produced.[6]
Wind energy
An estimated 1 to 3% of energy from the Sun that hits the earth is converted into wind energy. This is about 50 to 100 times more energy than is converted into biomass by all the plants on earth through photosynthesis. Most of this wind energy can be found at high altitudes where continuous wind speeds of over 160 km/h (100 mph) occur. Eventually, the wind energy is converted through friction into diffuse heat all through the earth's surface and atmosphere.
The origin of wind is simple. The earth is unevenly heated by the sun resulting in the poles receiving less energy from the sun than the equator does. Also the dry land heats up (and cools down) more quickly than the seas do. The differential heating powers a global atmospheric convection system reaching from the earth's surface to the stratosphere which acts as a virtual ceiling.
The change of seasons, change of day and night, the Coriolis effect, the irregular albedo (reflectivity) of land and water, humidity, and the friction of wind over different terrain are some of the factors which complicate the flow of wind over the surface.
Wind variability and turbine power
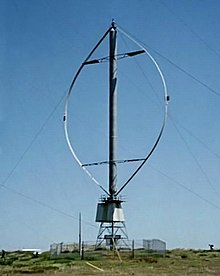
The power in the wind can be extracted by allowing it to blow past moving wings that exert torque on a rotor. The amount of power transferred is directly proportional to the density of the air, the area swept out by the rotor, and the cube of the wind speed.
The mass flow of air that travels through the swept area of a wind turbine varies with the wind speed and air density. As an example, on a cool 15°C (59°F) day at sea level, air density is about 1.22 kilograms per cubic metre (it gets less dense with higher humidity). An 8 m/s breeze blowing through a 100 meter diameter rotor would move about 76,000 kilograms of air per second through the swept area.
The kinetic energy of a given mass varies with the square of its velocity. Because the mass flow increases linearly with the wind speed, the wind energy available to a wind turbine increases as the cube of the wind speed. The power of the example breeze above through the example rotor would be about 2.5 megawatts.
As the wind turbine extracts energy from the air flow, the air is slowed down, which causes it to spread out and diverts it around the wind turbine to some extent. A German physicist, Albert Betz, determined in 1919 that a wind turbine can extract at most 59% of the energy that would otherwise flow through the turbine's cross section. The Betz limit applies regardless of the design of the turbine. More recent work by Gorlov shows a theoretical limit of about 30% for propeller-type turbines.[7] Actual efficiencies range from 10% to 20% for propeller-type turbines, and are as high as 35% for three-dimensional vertical-axis turbines like Darrieus or Gorlov turbines.

Windiness varies, and an average value for a given location does not alone indicate the amount of energy a wind turbine could produce there. To assess the climatology of wind speeds at a particular location, a probability distribution function is often fit to the observed data. Different locations will have different wind speed distributions. The distribution model most frequently used to model wind speed climatology is a two-parameter Weibull distribution because it is able to conform to a wide variety of distribution shapes, from gaussian to exponential. The Rayleigh model, an example of which is plotted to the right against an actual measured dataset, is a specific form of the Weibull function in which the shape parameter equals 2, and very closely mirrors the actual distribution of hourly wind speeds at many locations.
Because so much power is generated by higher windspeed, much of the average power available to a windmill comes in short bursts. The 2002 Lee Ranch sample is telling: half of the energy available arrived in just 15% of the operating time. The consequence of this is that wind energy is not dispatchable as for fuel-fired power plants; additional output cannot be supplied in response to load demand.
Since wind speed is not constant, a wind generator's annual energy production is never as much as its nameplate rating multiplied by the total hours in a year. The ratio of actual productivity in a year to this theoretical maximum is called the capacity factor. A well-sited wind generator will have a capacity factor of as much as 35%. When comparing the size of wind turbine plants to fueled power plants, it is important to note that 1000 kW of wind-turbine potential power would be expected to produce as much energy in a year as approximately 350 kW of fuel-fired generation. Though the short-term (hours or days) output of a wind-plant is not completely predictable, the annual output of energy tends to vary only a few percent points between years.
When storage, such as with pumped hydroelectric storage, or other forms of generation are used to "shape" wind power (by assuring constant delivery reliability), commercial delivery represents a cost increase of about 25%, yielding viable commercial performance.[1]
Wind power density classes
Wind maps in the United States and Europe classify areas into seven classes of wind power density, which give an indication of the quality of wind power resource in the area.
Each class is a range of power densities, so that an area rated as class 4, for example, would have an average power density from 200 to 250 W/m2 at 10 m above ground. Generally, economic development of wind power for electricity generation takes place in areas rated Class 3 or higher.
Wind Power Class |
10 m (33 ft) | 50 m (164 ft) | ||
---|---|---|---|---|
Wind power density (W/m2) | Speed[10] m/s (mph) | Wind power density (W/m2) | Speed[10] m/s (mph) | |
1 | 0 | 0 | 0 | 0 |
100 | 4.4 (9.8) | 200 | 5.6 (12.5) | |
2 | ||||
150 | 5.1 (11.5) | 300 | 6.4 (14.3) | |
3 | ||||
200 | 5.6 (12.5) | 400 | 7.0 (15.7) | |
4 | ||||
250 | 6.0 (13.4) | 500 | 7.5 (16.8) | |
5 | ||||
300 | 6.4 (14.3) | 600 | 8.0 (17.9) | |
6 | ||||
400 | 7.0 (15.7) | 800 | 8.8 (19.7) | |
7 | ||||
1000 | 9.4 (21.1) | 2000 | 11.9 (26.6) |
Turbine siting
As a general rule, wind generators are practical where the average wind speed is greater than 20 km/h (5.5 m/s or 12.5 mph). Obviously, meteorology plays an important part in determining possible locations for wind parks, though it has great accuracy limitations. Meteorological wind data is not usually sufficient for accurate siting of a large wind power project. An 'ideal' location would have a near constant flow of non-turbulent wind throughout the year and would not suffer too many sudden powerful bursts of wind.
The wind blows faster at higher altitudes because of the reduced influence of drag of the surface (sea or land) and the reduced viscosity of the air. The increase in velocity with altitude is most dramatic near the surface and is affected by topography, surface roughness, and upwind obstacles such as trees or buildings. Typically, the increase of wind speeds with increasing height follows a logarithmic profile that can be reasonably approximated by the wind profile power law, using an exponent of 1/7th, which predicts that wind speed rises proportionally to the seventh root of altitude. Doubling the altitude of a turbine, then, increases the expected wind speeds by 10% and the expected power by 34%.
Wind farms or wind parks often have many turbines installed. Since each turbine extracts some of the energy of the wind, it is important to provide adequate spacing between turbines to avoid excess energy loss. Where land area is sufficient, turbines are spaced three to five rotor diameters apart perpendicular to the prevailing wind, and five to ten rotor diameters apart in the direction of the prevailing wind, to minimize efficiency loss. The "wind park effect" loss can be as low as 2% of the combined nameplate rating of the turbines.
Utility-scale wind turbine generators have low temperature operating limits which restrict the application in areas that routinely experience temperatures less than −20 °C. Wind turbines must be protected from ice accumulation, which can make anemometer readings inaccurate and which can cause high structure loads and damage. Some turbine manufacturers offer low-temperature packages at a few percent extra cost, which include internal heaters, different lubricants, and different alloys for structural elements, to make it possible to operate the turbines at lower temperatures. If the low-temperature interval is combined with a low-wind condition, the wind turbine will require station service power, equivalent to a few percent of its output rating, to maintain internal temperatures during the cold snap. For example, the St. Leon, Manitoba project has a total rating of 99 MW and is estimated to need up to 3 MW (around 3% of capacity) of station service power a few days a year for temperatures down to −30 °C. This factor affects the economics of wind turbine operation in cold climates.[1]
Rural communities are thought to welcome wind farms because they provide income to farmers and ranchers, skilled jobs, cheap electricity and additional tax revenue to upgrade schools and maintain roads.
Onshore
Onshore turbine installations tend to be along mountain ridges or passes, or at the top of cliff faces. The change in ground elevation causes the wind velocities to be generally higher in these areas, although there may be variation over short distances (a difference of 30 m can sometimes mean a doubling in output). Local winds are often monitored for a year or more with anemometers and detailed wind maps constructed before wind generators are installed.
For smaller installations where such data collection is too expensive or time consuming, the normal way of prospecting for wind-power sites is to directly look for trees or vegetation that are permanently "cast" or deformed by the prevailing winds. Another way is to use a wind-speed survey map, or historical data from a nearby meteorological station, although these methods are less reliable.
Sea shores also tend to be windy areas and good sites for turbine installation, because a primary source of wind is convection from the differential heating and cooling of land and sea over the course of day and night. Winds at sea level carry somewhat more energy than winds of the same speed in mountainous areas because the air at sea level is more dense.
Unfortunately, windy areas tend to be picturesque, and so there is sometimes opposition to the installation of wind turbines on what would otherwise be ideal sites.
Offshore

Offshore wind turbines are considered to be less unsightly since they can be invisible from shore. Because there are fewer obstacles and stronger winds, such turbines don´t need to be built as high into the air. However, offshore conditions are harsh, abrasive, and corrosive, and it is more difficult to maintain a turbine in open waters than on land.
In areas with extended shallow continental shelves and sand banks (such as Denmark), turbines are reasonably easy to install, and give good service. At the site shown, the wind is not especially strong but is very consistent. The largest offshore wind turbines in the world are seven 3.6 MW rated machines off the east coast of Ireland about 60 km south of Dublin. The turbines are located on a sandbank approximately 10 km from the coast that has the potential for the installation of 500 MW of generation capacity. As of 2006, the largest offshore wind farm is Horns Rev which is located 15 km west off the West coast of Jutland, Denmark and produces 160 MW of power.[11]
Airborne
It has been suggested that wind turbines might be flown in high speed winds at high altitude. No such systems currently exist in the marketplace. An Ontario company, Magenn Power, Inc., is attempting to commercialize tethered aerial turbines suspended with helium.
Utilization
Large scale
Total installed windpower capacity (end of year & latest estimates)[12] | ||||
---|---|---|---|---|
Capacity (MW) | ||||
Rank | Nation | 03/2006 | 2005 | 2004 |
1 | Germany | 18,428 | 16,629 | |
2 | Spain | 10,027 | 8,263 | |
3 | USA | 9,549 | 9,149 | 6,725 |
4 | India | 5,200 | 4,430 | 3,000 |
5 | Denmark | 3,128 | 3,124 | |
6 | Italy | 1,717 | 1,265 | |
7 | United Kingdom | 1,598 | 1,353 | 888 |
8 | China | 1,260 | 764 | |
9 | Netherlands | 1,219 | 1,078 | |
10 | Japan | 1,040 | 896 | |
11 | Portugal | 1,022 | 522 | |
12 | Austria | 819 | 606 | |
13 | France | 918 | 757 | 386 |
14 | Canada | 943 | 683 | 444 |
15 | Greece | 573 | 473 | |
16 | Australia | 572 | 379 | |
17 | Sweden | 510 | 452 | |
18 | Ireland | 496 | 339 | |
19 | Norway | 270 | 270 | |
20 | New Zealand | 168 | 168 | |
21 | Belgium | 167 | 95 | |
22 | Egypt | 145 | 145 | |
23 | South Korea | 119 | 23 | |
24 | Taiwan | 103 | 13 | |
25 | Finland | 82 | 82 | |
26 | Poland | 73 | 63 | |
27 | Ukraine | 73 | 69 | |
28 | Costa Rica | 70 | 70 | |
29 | Morocco | 64 | 54 | |
30 | Luxembourg | 35 | 35 | |
31 | Iran | 32 | 25 | |
32 | Estonia | 30 | 3 | |
33 | Philippines | 29 | 29 | |
34 | Brazil | 29 | 24 | |
35 | Czech Republic | 28 | 17 | |
World total | ~60,100 | 58,982 | 47,671 |
There are many thousands of wind turbines operating, with a total capacity of 58,982 MW of which Europe accounts for 69% (2005). It was the most rapidly-growing means of alternative electricity generation at the turn of the century and provides a valuable complement to large-scale base-load power stations. World wind generation capacity more than quadrupled between 1999 and 2005. 90% of wind power installations are in the US and Europe, but the share of the top five countries in terms of new installations fell from 71 % in 2004 to 55 % in 2005. By 2010, World Wind Energy Association expects 120,000 MW to be installed worldwide.[12]
Germany, Spain, the United States, India and Denmark have made the largest investments in wind generated electricity. Denmark is prominent in the manufacturing and use of wind turbines, with a commitment made in the 1970s to eventually produce half of the country's power by wind. Denmark generates over 20% of its electricity with wind turbines, the highest percentage of any country and is fifth in the world in total power generation. Denmark and Germany are leading exporters of large (0.66 to 5 MW) turbines.
Wind accounts for 1% of the total electricity production on a global scale (2005). Germany is the leading producer of wind power with 32% of the total world capacity in 2005 (6% of German electricity); the official target is that by 2010, renewable energy will meet 12.5% of German electricity needs - it can be expected that this target will be reached even earlier. Germany has 16,000 wind turbines, mostly in the north of the country - including three of the biggest in the world, constructed by the companies Enercon (4.5 MW), Multibrid (5 MW) and Repower (5 MW). Germany's Schleswig-Holstein province generates 25% of its power with wind turbines.
Spain and the United States are next in terms of installed capacity. In 2005, the government of Spain approved a new national goal for installed wind power capacity of 20,000 MW by 2012. According to the American Wind Energy Association, wind generated enough electricity to power 0.4% (1.6 million households) of total electricity in US, up from less than 0.1% in 1999. In 2005, both Germany and Spain have produced more electricity from wind power than from hydropower plants. US Department of Energy studies have concluded wind harvested in just three of the fifty U.S. states could provide enough electricity to power the entire nation, and that offshore wind farms could do the same job.[1] Wind power could grow by 50% in the U.S. in 2006.[13]
India ranks 4th in the world with a total wind power capacity of 4,430 MW. Wind power generates 3% of all electricity produced in India. The World Wind Energy Conference in New Delhi in November 2006 will give additional impetus to the Indian wind industry.[12] In December 2003, General Electric installed the world's largest offshore wind turbines in Ireland, and plans are being made for more such installations on the west coast, including the possible use of floating turbines.
On August 15, 2005, China announced it would build a 1000-megawatt wind farm in Hebei for completion in 2020. China reportedly has set a generating target of 20,000 MW by 2020 from renewable energy sources - it says indigenous wind power could generate up to 253,000 MW. Following the World Wind Energy Conference in November 2004, organised by the Chinese and the World Wind Energy Association, a Chinese renewable energy law was adopted. In late 2005, the Chinese government increased the official wind energy target for the year 2020 from 20 GW to 30 GW.
Another growing market is Brazil, with a wind potential of 143 GW.[14] The federal government has created an incentive program, called Proinfa,[15] to build production capacity of 3300 MW of renewable energy for 2008, of which 1422 MW through wind energy. The program seeks to produce 10% of Brazillian electricity through renewable sources. Brazil produced 320 TWh in 2004.
Recently, Canada has experienced rapid growth of wind capacity - moving from a total installed capacity of 137MW to 943MW in under 6 years, showing a growth rate of 38% from 2000-2005, and rising.[16] This growth has been fed by provincial measures, including installation targets, economic incentives and political support. For example, the government of the Canadian province of Ontario announced on 21 March 2006 that it will introduce a feed-in tariff for wind power, referred to as 'Standard Offer Contracts', which may boost the wind industry across the entire country.[17] In the Canadian province of Quebec, the state-owned hydroelectric utility plans to generate 2000 MW from wind farms by 2013.[18]
Small scale

Wind turbines have been used for household electricity generation in conjunction with battery storage over many decades in remote areas. Household generator units of more than 1 kW are now functioning in several countries.
To compensate for the varying power output, grid-connected wind turbines may utilise some sort of grid energy storage. Off-grid systems either adapt to intermittent power or use photovoltaic or diesel systems to supplement the wind turbine.
Wind turbines range from small four hundred watt generators for residential use to several megawatt machines for wind farms and offshore. The small ones have direct drive generators, direct current output, aeroelastic blades, lifetime bearings and use a vane to point into the wind; while the larger ones generally have geared power trains, alternating current output, flaps and are actively pointed into the wind. Direct drive generators and aeroelastic blades for large wind turbines are being researched and direct current generators are sometimes used.
In urban locations, where it is difficult to obtain large amounts of wind energy, smaller systems may still be used to run low power equipment. Distributed power from rooftop mounted wind turbines can also alleviate power distribution problems, as well as provide resilience to power failures. Equipment such as parking meters or wireless internet gateways may be powered by a wind turbine that charges a small battery, replacing the need for a connection to the power grid and/or maintaining service despite possible power grid failures.

Small scale turbines are available that are approximately 7 feet (2 m) in diameter and produce 900 watts. Units are lightweight, e.g. 16 kg (35 pounds), allowing rapid respond to wind gusts typical of urban settings and easy mounting much like a television antenna. It is claimed that they are inaudible even a few feet under the turbine. Dynamic braking regulates the speed by dumping excess energy, so that the turbine continues to produce electricity even in high winds. The dynamic braking resistor may be installed inside the building to provide heat (during high winds when more heat is lost by the building, while more heat is also produced by the braking resistor). The proximal location makes low voltage (12 volt, or the like) energy distribution practical. An additional benefit is that owners become more aware of electricity consumption, possibly reducing their consumption down to the average level that the turbine can produce.
According to the World Wind Energy Association, it is difficult to assess the total number or capacity of small-scaled wind turbines, but in China alone, there are roughly 300,000 small-scale wind turbines generating electricity.[12]
Controversy
Arguments for and against wind power are listed below.
Arguments of opponents

Economics
- To compete with traditional sources of energy, wind power often receives financial incentives. In the United States, wind power receives a tax credit of 1.9 cents per kilowatt-hour produced, with a year inflationary adjustment. However, in 2004 when the U.S. production tax credit had lapsed for nine months, wind power was still a rapidly growing form of electrical generation. Another tax benefit is accelerated depreciation. Many American states also provide incentives, such as exemption from property tax, mandated purchases, and additional markets for "green credits." Countries such as Canada and Germany also provide tax credits and other incentives for wind turbine construction.
- Maintenance of wind turbines can be difficult and expensive. Repairs require a much more complicated and expensive operation than ground based generation.
- Many potential sites for wind farms are far from demand centers, requiring substantially more money to construct new transmission lines and substations.
Yield
- The goals of renewable energy development are reduction of reliance on fossil and nuclear fuels, reduction of greenhouse gas and other emissions, and establishment of more sustainable sources of energy. Some critics question wind energy's ability to significantly move society towards these goals. They point out that 25-30% annual load factor is typical for wind facilities. The intermittent and non-dispatchable nature of wind turbine power requires that "spinning reserves" are kept burning for supply security. The fluctuation in wind power requires more frequent load ramping of such plant to maintain grid system frequency. This can force operators to run conventional plant below optimal thermal efficiency resulting in greater emissions. A recent European Nuclear Society study estimates that the equivalent of one third of energy saved from wind generation is lost to these inefficiencies.
CO2 Emissions
- Electric power production is only part (about one to two fifths[19]) of a country's energy use, and wind power does nothing to mitigate the larger part of the effects of energy use. For example, despite aggressive installation of wind facilities in the U.K., that country's CO2 emissions continued to rise in 2002 and 2003 (Department of Trade and Industry). Six of the U.K.'s nuclear reactors were closed in this period.[20]
- Groups such as the UN's Intergovernmental Panel on Climate Change state that the desired mitigation goals can be achieved at lower cost and to a greater degree by continued improvements in general efficiency — in building, manufacturing, and transport — than by wind power. Such statements, however, do not take into account long-term costs and calculations, like drastically increasing prices for oil, gas, uranium etc. Also once an investment in a wind turbine is made, the electricity produced by that turbine is fixed for a period of 20 years.
Ecological footprint
- The construction of a large facility is also far from ecologically neutral if the location has no previous development. It requires roads, foundations, clearing of trees, and construction of power lines. The clearing of trees may be necessary since obstructions within a distance ten times the height of the turbine reduce yield dramatically. A distance of twenty times is preferred.
- While it might be true that during normal operation a windfarm does not emit any greenhouse gases such as carbon dioxide or other air pollution (such as PM10 soot) it is important to consider the entire lifecycle of the wind turbine. To create a wind machine, steel, concrete, aluminium and other materials will have to be made and transported (using for example diesel). The energy, externality, and economic payback period of a wind turbine lies between four and six months, depending on the energy yield.
- A wind farm that produces the energy equivalent of a conventional power plant would have to cover an area of approximately 200 square kilometres. [citation needed]
- Offshore sites have on average a higher energy yield than onshore sites.
- Windmills kill birds, especially birds of prey. Siting generally takes into account known bird flight patterns, but most paths of bird migration, particularly for birds that fly by night, are unknown. Although a Danish survey in 2005 (Biology Letters 2005:336) showed that less than 1% of migrating birds passing a wind farm in Rønde, Denmark, got close to collision, the site was studied only during low-wind non-twilight conditions. A survey at Altamont Pass, California conducted by a California Energy Commission in 2004 showed that turbines killed 4,700 birds annually (1,300 of which are birds of prey). Radar studies of proposed sites in the eastern U.S. have shown that migrating songbirds fly well within the reach of large modern turbines.
- The numbers of bats killed by existing facilities has troubled even industry personnel.[21] A six-week study in 2004 estimated that over 2200 bats were killed by 63 turbines at two sites in the Eastern US.[22] This study suggests some site locations may be particularly hazardous to local bat populations, and that more research is urgently needed.
Scalability
- To meet the energy demands worldwide in the future in a sustainable way, a much larger number of turbines than today will be required. Naturally this will affect more people and wildlife habitat.
Aesthetics
- Perceptions that wind turbines are noisy and contribute to "visual pollution" creates resistance to the establishment of land-based wind farms in some places. Moving the turbines offshore mitigates the problem, but offshore wind farms are more expensive to maintain and there is an increase in transmission loss due to longer distances of power lines. One solution to such objections is the early and close involvement of the local population, like recommended in the sustainability guidelines of the World Wind Energy Association[12] - in the ideal case through community/citizen ownership of wind farms.
- Some residents near windmills complain of "shadow flicker," which is the alternating pattern of sun and shade caused by a rotating windmill casting a shadow over residences. Efforts are made when siting turbines to avoid this problem.
Arguments of supporters

Supporters of wind energy state that:
Pollution
- Wind power is a renewable resource, which means using it will not deplete the earth's supply of fossil fuels. It also is a clean energy source, and does not directly produce carbon dioxide, sulfur dioxide, mercury, particulates, or any other type of air pollution, as do conventional fossil fuel power sources. Indirectly, carbon dioxide and other types of air pollution are released when materials for construction are mined and processed, but that holds true for every other power plant type. Unlike nuclear power, wind power does not produce any radioactive waste.
Long-term potential
- Wind's long-term technical potential is believed 5 times current global energy consumption or 40 times current electricity demand. This requires 12.7% of all land area, or that land area with Class 3 or greater potential at a height of 80 meters. It assumes that the land is covered with 6 large wind turbines per square kilometer. Offshore resources experience mean wind speeds ~90% greater than that of land, so offshore resources could contribute ~7 times more energy than land.[23][24] This number could also increase with higher altitude or airborne wind turbines.[25]
Coping with intermittency
- As the fraction of energy produced by wind ("penetration") increases, different technical and economic factors affect the need, if there is one, for grid energy storage facilities. Large networks, connected to multiple wind plants at widely separated geographic locations, may accept a higher penetration of wind than small networks or those without storage systems or economical methods of compensating for the variability of wind. In systems with significant amounts of existing pumped storage (e.g. UK, eastern US) this proportion may be higher. Isolated, relatively small systems with only a few wind plants may only be stable and economic with a lower fraction of wind energy (e.g. Ireland).
- On most large power systems a moderate proportion of wind generation can be connected without the need for storage. For larger proportions, storage may be economically attractive or even technically necessary.
- Long-term storage of electrical energy involves substantial capital costs, space for storage facilities, and some portion of the stored power will be lost during conversion and transmission. The percentage retrievable from stored power is called the "efficiency of storage." The cost incurred to "shape" intermittent wind power for reliable delivery is about a 20% premium for most wind applications on large grids, but approaches 50% of the cost of generation when wind comprises more than 70% of the local grid's input power. See: Grid energy storage
- Electricity demand is variable but generally very predictable on larger grids; errors in demand forecasting are typically no more than 2%. Because conventional powerplants can drop off the grid within a few seconds, for example due to equipment failures, in most systems the output of some coal or gas powerplants is intentionally part-loaded to follow demand and to replace rapidly lost generation. The ability to follow demand (by maintaining constant frequency) is termed "response." The ability to quickly replace lost generation, typically within timescales of 30 seconds to 30 minutes, is termed "spinning reserve." Nuclear power plants in contrast are not very flexible and are not intentionally part-loaded. A power plant that operates in a steady fashion, usually for many days continuously, is termed a "base load" plant.
- What happens in practice therefore is that as the power output from wind varies, part-loaded conventional plants, which must be there anyway due to changing demand, adjust their output to compensate; they do this in response to small changes in the frequency (nominally 50 or 60 Hz) of the grid. In this sense wind acts like "negative" load or demand.
- The maximum proportion of wind power allowable in a power system will thus depend on many factors, including the size of the system, the attainable geographical diversity of wind, the conventional plant mix (coal, gas, nuclear) and seasonal load factors (heating in winter, air-conditioning in summer) and their statistical correlation with wind output. For most large systems the allowable penetration fraction (wind nameplate rating divided by system peak demand) is thus at least 15% without the need for any energy storage whatsoever. Note that the interconnected electrical system may be much larger than the particular country or state (e.g. Denmark, California) being considered.
- It should also be borne in mind that wind output, especially from large numbers of turbines/farms can be predicted with a fair degree of confidence many hours ahead using weather forecasts.
- The allowable penetration may of course be further increased by increasing the amount of part-loaded generation available, or by using energy storage facilities, although if purpose-built for wind energy these may significantly increase the overall cost of wind power.
- Existing European hydroelectric power plants can store enough energy to supply one month's worth of European electricity consumption. Improvement of the international grid would allow using this in the relatively short term at low cost, supplementing wind power. Excess wind power could even be used to pump water up into collection basins for later use.
- Energy Demand Management or Demand-Side Management refers to the use of communication and switching devices which can release deferrable loads quickly to correct supply/demand imbalances. Incentives can be created for the use of these systems, such as favorable rates or capital cost assistance, encouraging consumers with large loads to take advantage of renewable energy by adjusting their loads to coincide with resource availability. For example, pumping water to pressurize municipal water systems is an electricity intensive application that can be performed when electricity is available.[26]
- In energy schemes with a high penetration of wind energy, secondary loads, such as desalination plants and electric boilers may be encouraged because their output (water and heat) can be stored. The utilization of "burst electricity", where excess electricity is used on windy days for opportunistic purposes greatly improves the economic efficiency of wind turbine schemes. An ice storage device has been invented which allows cooling energy to be consumed during resource availability, and dispatched as air conditioning during peak hours.
- Multiple wind farms spread over a wide geographic area and gridded together produce power much more constantly.
- Electricity produced from solar energy could be a counter balance to the fluctuating supplies generated from wind. It tends to be windier at night and during cloudy or stormy weather, so there is likely to be more sunshine when there is less wind.
Ecology
- Because it uses energy already present in the atmosphere, and can displace fossil-fuel generated electricity (with its accompanying carbon dioxide emissions), wind power mitigates global warming. If the entire world's nameplate electrical demand expected in 2010 were served from wind power alone, the amount of energy extracted from the atmosphere would be less than the increase added by radiative forcing by additional carbon dioxide at 2000 levels above those of the year 1500, before fossil fuel consumption became significant.[citation needed]
- Energy payback ratio (ratio of energy produced compared to energy expended in construction and operation)for wind turbines is between 17 and 39 (i.e. over it's life-time a wind turbine produces 17-39 times as much energy as is needed for its manufacture, construction, operation and decomissioning). This is to be compared with 11 for coal power plants and 16 for nuclear power plants.[27]
- The energy consumption for production, installation, operation and decommissioning of a wind turbine is usually earned back within 3 months of operation.[28]
- Unlike fossil or nuclear power stations, which circulate large amounts of water for cooling, wind turbines do not need water to generate electricity.
- Studies show that the number of birds killed by wind turbines is negligible compared to the amount that die as a result of other human activities such as traffic, hunting, power lines and high-rise buildings and especially the environmental impacts of using non-clean power sources. For example, in the UK, where there are a few hundred turbines, about one bird is killed per turbine per year; 10 million per year are killed by cars alone.[29] Another study suggests that migrating birds adapt to obstacles; those birds which don't modify their route and continue to fly through a wind farm are capable of avoiding windmills,[30] at least in the low-wind non-twilight conditions studied. In the UK, the Royal Society for the Protection of Birds (RSPB) concluded that "The available evidence suggests that appropriately positioned wind farms do not pose a significant hazard for birds."[31] It notes that climate change poses a much more significant threat to wildlife, and therefore supports wind farms and other forms of renewable energy.
- Clearing of wooded areas is often unnecessary, as the practice of farmers leasing their land out to companies building wind farms is common. Farmers receive annual lease payments of two thousand to five thousand dollars per turbine.[32] The land can still be used for farming and cattle grazing.
- The ecological and environmental costs of wind plants are paid by those using the power produced, with no long-term effects on climate or local environment left for future generations.
- Less than 1% of the land would be used for foundations and access roads, the other 99% could still be used for farming.[33] Turbines can be sited on land unused in techniques such as center-pivot irrigation.
- After decommissioning wind turbines, even the foundations are removed.
Economic feasibility
- Conventional and nuclear power plants receive massive amounts of direct and indirect governmental subsidies. If a comparison is made on real production costs, wind energy is competitive in many cases. If the full costs (environmental, health, etc.) are taken into account, wind energy is competitive in most cases. Furthermore, wind energy costs are continuously decreasing due to technology development and scale enlargement.
- Nuclear power plants receive special immunity from the disasters they may cause, which prevents victims from recovering the cost of their continued health care from those responsible, even in the case of criminal malfeasance.
- Conventional and nuclear plants also have sudden unpredictable outages (see above). Statistical analysis shows that 1000 MW of wind power can replace 300 MW of conventional power.
Aesthetics

- Improvements in blade design and gearing have quietened modern turbines to the point where a normal conversation can be held underneath one
- Newer wind farms have more widely spaced turbines due to the greater power of the individual wind turbines, and so look less cluttered
- Wind turbines can be positioned alongside motorways, significantly reducing aesthetic concerns
- The aesthetics of wind turbines have been compared favourably to those of pylons from conventional power stations
- Areas under windfarms can be used for farming, and are protected from development
See also
Power Generation
- Fossil fuel power and petro-free
- Hydropower
- Nuclear Power
- Solar power and nuclear residues.
- Steam engine.
Green Energy
- Green energy
- Template:user green energy
- Green tax shift
- Grid energy storage
- Renewable energy
- Nuclear power phase-out
- Wind
- Windmill
- Wind farm
- Wind turbine
References
- ^ a b c Mitchell, Chris (March 23, 2006). "Price of Wind-Generated Electricity Plummeting". Retrieved 2006-04-21.
{{cite web}}
: CS1 maint: year (link) - ^ Chakrabarty, Gargi (March 27, 2004). "Powering up". Rocky Mountain News. Retrieved 2004-04-05.
{{cite web}}
: CS1 maint: year (link) (Internet Archive version) - ^ "E-Letter responses to: The Real Cost of Wind Energy". Science. Retrieved 2006-04-21.
- ^ "Alternate Power: A Change Is In The Wind". Business Week. July 4, 2005. Retrieved 2006-04-21.
{{cite web}}
: CS1 maint: year (link) - ^ "Renewable Energy Trends 2003" (PDF). DOE/EIA. July 2004. Retrieved 2006-04-21.
{{cite web}}
: CS1 maint: year (link) - ^ Helming, Troy (February 2, 2004). "Uncle Sam's New Year's Resolution". Retrieved 2006-04-21.
{{cite web}}
: Unknown parameter|publsiher=
ignored (|publisher=
suggested) (help)CS1 maint: year (link) - ^ Gorban, Alexander N. (2001). "Limits of the Turbine Efficiency for Free Fluid Flow" (PDF). Journal of Energy Resources Technology. 123: 311–317. Retrieved 2006-04-21.
{{cite journal}}
: Unknown parameter|month=
ignored (help) - ^ Vertical extrapolation of wind speed based on the 1/7 power law.
- ^ "Wind Energy Resource Atlas of the United States". Renewable Resource Data Center. Retrieved 2006-04-21.
- ^ a b Mean wind speed is based on Rayleigh speed distribution of equivalent mean wind power density. Wind speed is for standard sea-level conditions. To maintain the same power density, speed increases 3%/1000 m (5%/5000 ft) elevation.
- ^ "Horns Rev offshore wind farm". Elsam. Retrieved 2006-04-21.
- ^ a b c d e "the World Wind Energy Association (WWEA) web site". Retrieved 2006-04-21.
- ^ Aslam, Abid (Mar. 31, 2006). "Problem: Foreign Oil, Answer: Blowing in the Wind?". OneWorld US. Retrieved 2006-04-21.
{{cite web}}
: Check date values in:|year=
(help)CS1 maint: year (link) - ^ "Atlas do Potencial Eólico Brasileiro". Retrieved 2006-04-21.
- ^ "Eletrobrás - Centrais Elétricas Brasileiras S.A - Projeto Proinfa". Retrieved 2006-04-21.
- ^ "Wind Energy: Rapid Growth" (PDF). Canadian Wind Energy Association. Retrieved 2006-04-21.
- ^ "Standard Offer Contracts Arrive In Ontario". Ontario Sustainable Energy Association. March 21, 2006. Retrieved 2006-04-21.
{{cite web}}
: CS1 maint: year (link) - ^ "Call for Tenders A/O 2005-03: Wind Power 2,000 MW". Hydro-Québec. Retrieved 2006-04-21.
- ^ "Annual Energy Review 2004 Report No. DOE/EIA-0384(2004)". Energy Information Administration. August 15, 2005. Retrieved 2006-04-21.
{{cite web}}
: CS1 maint: year (link) - ^ "Nuclear Power in the United Kingdom - Briefing Paper # 84". Uranium Information Centre Ltd. December 2005. Retrieved 2006-04-21.
{{cite web}}
: CS1 maint: year (link) - ^ "Caution Regarding Placement of Wind Turbines on Wooded Ridge Tops" (PDF). Bat Conservation International. 4 January 2005. Retrieved 2006-04-21.
{{cite web}}
: CS1 maint: year (link) - ^ Arnett, Edward B. (June 2005). "Relationships between Bats and Wind Turbines in Pennsylvania and West Virginia: An Assessment of Fatality Search Protocols, Patterns of Fatality, and Behavioral Interactions with Wind Turbines" (PDF). Bat Conservation International. Retrieved 2006-04-21.
{{cite web}}
: Unknown parameter|coauthors=
ignored (|author=
suggested) (help)CS1 maint: year (link) - ^ Archer, Cristina L. "Evaluation of global wind power". Retrieved 2006-04-21.
{{cite web}}
: Unknown parameter|coauthors=
ignored (|author=
suggested) (help) - ^ "Global Wind Map Shows Best Wind Farm Locations". Environment News Service. May 17, 2005. Retrieved 2006-04-21.
{{cite web}}
: CS1 maint: year (link) - ^ Cohn, David (Apr 06, 2005). "Windmills in the Sky". Wired News. Retrieved 2006-04-21.
{{cite web}}
: Check date values in:|year=
(help)CS1 maint: year (link) - ^ "2005 Integrated Energy Policy Report". California Energy Commission. November 21, 2005. Retrieved 2006-04-21.
{{cite web}}
: CS1 maint: year (link) - ^ "Net Energy Payback and CO2 Emissions from Wind-Generated Electricity in the Midwest" (PDF). S.W.White & G.L.Klucinski - Fusion Technology Institute University of Wisconsin. December 1998. Retrieved 2006-05-12.
{{cite web}}
: CS1 maint: year (link) - ^ "Danish Wind Industry Association". Danis Wind Turbine Manufacturer's Association. December 1997. Retrieved 2006-05-12.
{{cite web}}
: CS1 maint: year (link) - ^ "404 error". Retrieved 2006-04-21.
{{cite web}}
: Cite uses generic title (help) - ^ "Wind turbines a breeze for migrating birds". New Scientist (2504): 21. 2005. Retrieved 2006-04-21.
{{cite journal}}
: Unknown parameter|month=
ignored (help) - ^ "Wind farms". Royal Society for the Protection of Birds. 14 September 2005. Retrieved 2006-04-21.
{{cite web}}
: CS1 maint: year (link) - ^ "RENEWABLE ENERGY - Wind Power's Contribution to Electric Power Generation and Impact on Farms and Rural Communities (GAO-04-756)" (PDF). United States Government Accountability Office. September 2004. Retrieved 2006-04-21.
{{cite web}}
: CS1 maint: year (link) - ^ "Wind energy Frequently Asked Questions". British Wind Energy Association. Retrieved 2006-04-21.
Sources
Technical
- Global wind power (includes map)
- Wind Power Impacts on Electric Power System Operating Costs by the U.S. National Renewable Energy Laboratory (2004)
- Wind Energy Projects and Daily News
- Average annual wind power map for the U.S.
- Wind Atlases of the World – The World of Wind Atlases
- Danish Organisation of wind-turbine manufactures Extensive technical information on windenergy.
- Homebrewed windenergy
- Discussion of application of wind turbines in cold climates
Political
- World Wind Energy Association WWEA
- German Wind Energy Association BWE
- Spanish Wind Energy Association (Asociación Empresarial Eólica)
- The British Wind Energy Association
- American Wind Energy Association
- African Wind Energy Association -- AfriWEA is a non-profit organisation formed in 2002 to encourage manufacturers, developers, governments, renewable energy owners and individuals to promote and support wind energy development on the African continent
- European Wind Energy Association Extensive information and myth debunking in the report: windenergy the facts.
- Canadian Wind Energy Association
- Alliance to Protect Nantucket Sound Citizens group opposed to plans for USA's first offshore wind facility.
- Capewind -- Website of USA's first offshore wind facility.
- Country Guardian -- A UK NGO opposed to the construction of wind turbines.
- National Wind Watch -- A US coalition of citizens and grassroots groups to promote knowledge and raise awareness of the damaging impacts of industrial wind turbine development.
- Industrial Wind Energy Opposition -- Resources for debunking claims, documenting ill effects, and fighting the spread of industrial wind power
- Gardner Mountain NH USA -- "A public community site" trying to stop some local wind turbine development.
- Iberica 2000.org - On the serious impact of wind farms on birds.
- Commentary on preceding paper - Birds have to fly farther to avoid windmills, fair-weather study finds.
- "We Must Increase Our Gust" - On wind power's ability to replace other sources, with lively forum discussion.
- "Shooting Down the Breeze" - On wind power industry's ambivalent concern about wildlife impacts, also with lively forum discussion.
- Wind Works --An extensive personal site on windpower. Nuanced pro wind energy.
- yes2wind -- A website by Greenpeace, Friends of the Earth and the WWF in support of wind energy and debunking claims of the anti-wind energy lobby.
- wind-farm -- A website for news, articles, and discussion of wind facilities.
- ExternE -- The European research project about the external costs of energy production in general.
- Wind systems - On the site of the Australian Greenhouse Office.
- Windpower Monthly -- Wind Energy Magazine.
- Enertrag.de -- A German energy company investing in wind turbines. Owns 13 wind parks as of October 2004.
- POWER - Pushing Offshore Wind Energy Regions -- POWER is an European Interreg project which establishes a discussion platform and a support of European regions in the development of offshore-windenergy projects.
Wind power projects
- Altamont Pass
- Austin Energy's GreenChoice Program
- Cape Wind (Massachusetts)
- Database of projects throughout the United States
- Judith Gap Wind Farm (Montana) now on-line
- Windiberica Wind Farm Projects (Spain)
- Mawson Station - High Penetration (>90%) Wind Diesel at Mawson Station in Antarctica.
- Denham - Wind Diesel in Western Australia.
- Wenerenergi Sweden
- Projects planned for Texas
Academic Institutions
External links
- UK's official Wind Farm website.
- A new gust of wind projects across the US High natural-gas prices and global-warming concerns may help wind energy gain critical mass. By Mark Clayton, Christian Science Monitor, January 19, 2006
- Green Mountain
- "Wind versus water: Measuring the potential of our renewable resources" from Canadian Geographic
- Climate Change Chronicles article about offshore wind energy in Europe
- Wind farms could meet energy needs
- Wind Power News Daily News about Wind Farms and Technology
- Wind Power Related News
- Wind power
- Wenerenergi
- Offshorewind.net -Offshore wind energy information.
- Energyorbit :: Towards Sustainable Power and Renewable Energy
- Sterling Planet