Transposable element: Difference between revisions
m Journal cites, using AWB (12158) |
No edit summary |
||
Line 2: | Line 2: | ||
[[File:Composite transposon.svg|thumb|right|350px|A bacterial DNA transposon]] |
[[File:Composite transposon.svg|thumb|right|350px|A bacterial DNA transposon]] |
||
A '''transposable element''' ('''TE''' or '''transposon''') is a [[DNA sequence]] that can change its position within a [[genome]], sometimes creating or reversing [[mutation]]s and altering the cell's [[genome size]]. Transposition often results in duplication of the TE. [[Barbara McClintock]]'s discovery of these '''jumping genes''' earned her a [[Nobel Prize]] in 1983.<ref>{{cite journal |last=McClintock |first=Barbara |date=June 1950 |title=The origin and behavior of mutable loci in maize |journal=Proc Natl Acad Sci U S A. |volume=36 |issue=6 |pages=344–55 |pmid=15430309 |doi=10.1073/pnas.36.6.344 |pmc=1063197 |bibcode=1950PNAS...36..344M}}</ref> |
A '''transposable element''' ('''TE''' or '''transposon''') is a [[DNA sequence]] that can change its position within a [[genome]], sometimes creating or reversing [[mutation]]s and altering the cell's genetic identity and [[genome size]]. Transposition often results in duplication of the TE. [[Barbara McClintock]]'s discovery of these '''jumping genes''' earned her a [[Nobel Prize]] in 1983.<ref>{{cite journal |last=McClintock |first=Barbara |date=June 1950 |title=The origin and behavior of mutable loci in maize |journal=Proc Natl Acad Sci U S A. |volume=36 |issue=6 |pages=344–55 |pmid=15430309 |doi=10.1073/pnas.36.6.344 |pmc=1063197 |bibcode=1950PNAS...36..344M}}</ref> |
||
Transposable elements make up a large fraction of the genome and are responsible for much of the [[C-value|mass of DNA]] in a [[eukaryotic cell]]. It has been shown that TEs are important in genome function and evolution.<ref>{{cite journal |vauthors=Bucher E, Reinders J, Mirouze M |date=Nov 2012 |title=Epigenetic control of transposon transcription and mobility in ''Arabidopsis'' |journal=Current Opinion in Plant Biology |volume=15 |issue=5 |pages=503–10 |pmid=22940592 |doi=10.1016/j.pbi.2012.08.006}}</ref> In [[Stichotrich|''Oxytricha'']], which has a unique genetic system, these elements play a critical role in development.<ref>{{cite web |url=http://www.sciencedaily.com/releases/2009/05/090520140408.htm |title='Junk' DNA Has Important Role, Researchers Find |work=Science Daily |date=21 May 2009}}</ref> Transposons are also very useful to researchers as a means to alter DNA inside a living organism. |
Transposable elements make up a large fraction of the genome and are responsible for much of the [[C-value|mass of DNA]] in a [[eukaryotic cell]]. It has been shown that TEs are important in genome function and evolution.<ref>{{cite journal |vauthors=Bucher E, Reinders J, Mirouze M |date=Nov 2012 |title=Epigenetic control of transposon transcription and mobility in ''Arabidopsis'' |journal=Current Opinion in Plant Biology |volume=15 |issue=5 |pages=503–10 |pmid=22940592 |doi=10.1016/j.pbi.2012.08.006}}</ref> In [[Stichotrich|''Oxytricha'']], which has a unique genetic system, these elements play a critical role in development.<ref>{{cite web |url=http://www.sciencedaily.com/releases/2009/05/090520140408.htm |title='Junk' DNA Has Important Role, Researchers Find |work=Science Daily |date=21 May 2009}}</ref> Transposons are also very useful to researchers as a means to alter DNA inside a living organism. |
Revision as of 11:25, 23 May 2017
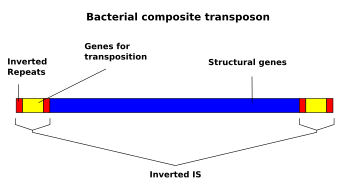
A transposable element (TE or transposon) is a DNA sequence that can change its position within a genome, sometimes creating or reversing mutations and altering the cell's genetic identity and genome size. Transposition often results in duplication of the TE. Barbara McClintock's discovery of these jumping genes earned her a Nobel Prize in 1983.[1]
Transposable elements make up a large fraction of the genome and are responsible for much of the mass of DNA in a eukaryotic cell. It has been shown that TEs are important in genome function and evolution.[2] In Oxytricha, which has a unique genetic system, these elements play a critical role in development.[3] Transposons are also very useful to researchers as a means to alter DNA inside a living organism.
There are at least two classes of TEs: Class I TEs or retrotransposons generally function via reverse transcription, while Class II TEs or DNA transposons encode the protein transposase, which they require for insertion and excision, and some of these TEs also encode other proteins.[4]
Discovery
Barbara McClintock discovered the first TEs in maize (Zea mays) at the Cold Spring Harbor Laboratory in New York. McClintock was experimenting with maize plants that had broken chromosomes.[5]
In the winter of 1944–1945, McClintock planted corn kernels that were self-pollinated, meaning that the silk (style) of the flower received pollen from its own anther.[5] These kernels came from a long line of plants that had been self-pollinated, causing broken arms on the end of their ninth chromosomes.[5] As the maize plants began to grow, McClintock noted unusual color patterns on the leaves.[5] For example, one leaf had two albino patches of almost identical size, located side by side on the leaf.[5] McClintock hypothesized that during cell division certain cells lost genetic material, while others gained what they had lost.[6] However, when comparing the chromosomes of the current generation of plants with the parent generation, she found certain parts of the chromosome had switched position.[6] This refuted the popular genetic theory of the time that genes were fixed in their position on a chromosome. McClintock found that genes could not only move, but they could also be turned on or off due to certain environmental conditions or during different stages of cell development.[6]
McClintock also showed that gene mutations could be reversed.[7] She presented her report on her findings in 1951, and published an article on her discoveries in Genetics in November 1953 entitled "Induction of Instability at Selected Loci in Maize."[8]
Her work would be largely dismissed and ignored until the late 1960s–1970s when it would be rediscovered after TEs were found in bacteria.[9] She was awarded a Nobel Prize in Physiology or Medicine in 1983 for her discovery of TEs, more than thirty years after her initial research.[10]
Approximately 90% of the maize genome is made up of TEs,[11] as is 44% of the human genome.[12]
Classification
Transposable elements represent one of several types of mobile genetic elements. TEs are assigned to one of two classes according to their mechanism of transposition, which can be described as either copy and paste (Class I TEs) or cut and paste (Class II TEs).[13]
Class I (retrotransposons)
Class I TEs are copied in two stages: first, they are transcribed from DNA to RNA, and the RNA produced is then reverse transcribed to DNA. This copied DNA is then inserted back into the genome at a new position. The reverse transcription step is catalyzed by a reverse transcriptase, which is often encoded by the TE itself. The characteristics of retrotransposons are similar to retroviruses, such as HIV.
Retrotransposons are commonly grouped into three main orders:
- TEs with long terminal repeats (LTRs), which encode reverse transcriptase, similar to retroviruses
- Long interspersed nuclear elements (LINEs, LINE-1s, or L1s), which encode reverse transcriptase but lack LTRs, and are transcribed by RNA polymerase II
- Short interspersed nuclear elements do not encode reverse transcriptase and are transcribed by RNA polymerase III
[Note : Retroviruses can also be considered TEs. For example, after conversion of retroviral RNA into DNA inside a host cell, the newly produced retroviral DNA is integrated into the genome of the host cell. These integrated DNAs are termed proviruses. The provirus is a specialized form of eukaryotic retrotransposon, which can produce RNA intermediates that may leave the host cell and infect other cells. The transposition cycle of retroviruses has similarities to that of prokaryotic TEs, suggesting a distant relationship between the two].
Class II (DNA transposons)
The cut-and-paste transposition mechanism of class II TEs does not involve an RNA intermediate. The transpositions are catalyzed by several transposase enzymes. Some transposases non-specifically bind to any target site in DNA, whereas others bind to specific target sequences. The transposase makes a staggered cut at the target site producing sticky ends, cuts out the DNA transposon and ligates it into the target site. A DNA polymerase fills in the resulting gaps from the sticky ends and DNA ligase closes the sugar-phosphate backbone. This results in target site duplication and the insertion sites of DNA transposons may be identified by short direct repeats (a staggered cut in the target DNA filled by DNA polymerase) followed by inverted repeats (which are important for the TE excision by transposase).
Cut-and-paste TEs may be duplicated if their transposition takes place during S phase of the cell cycle, when a donor site has already been replicated but a target site has not yet been replicated.[citation needed] Such duplications at the target site can result in gene duplication, which plays an important role in genomic evolution.[14]: 284
Not all DNA transposons transpose through the cut-and-paste mechanism. In some cases, a replicative transposition is observed in which a transposon replicates itself to a new target site (e.g. helitron).
Class II TEs comprise less than 2% of the human genome, making the rest Class I.[15]
Autonomous and non-autonomous
Transposition can be classified as either "autonomous" or "non-autonomous" in both Class I and Class II TEs. Autonomous TEs can move by themselves, whereas non-autonomous TEs require the presence of another TE to move. This is often because dependent TEs lack transposase (for Class II) or reverse transcriptase (for Class I).
Activator element (Ac) is an example of an autonomous TE, and dissociation elements (Ds) is an example of a non-autonomous TE. Without Ac, Ds is not able to transpose.
Examples
- The first TEs were discovered in maize (Zea mays) by Barbara McClintock in 1948, for which she was later awarded a Nobel Prize. She noticed chromosomal insertions, deletions, and translocations caused by these elements. These changes in the genome could, for example, lead to a change in the color of corn kernels. About 85% of the maize genome consists of TEs.[16] The Ac/Ds system described by McClintock are Class II TEs. Transposition of Ac in tobacco has been demonstrated by B. Baker (Plant Transposable Elements, pp 161–174, 1988, Plenum Publishing Corp., ed. Nelson).
- One family of TEs in the fruit fly Drosophila melanogaster are called P elements. They seem to have first appeared in the species only in the middle of the twentieth century; within the last 50 years, they spread through every population of the species. Gerald M. Rubin and Allan C. Spradling pioneered technology to use artificial P elements to insert genes into Drosophila by injecting the embryo.[17][18][19]
- Transposons in bacteria usually carry an additional gene for functions other than transposition, often for antibiotic resistance. In bacteria, transposons can jump from chromosomal DNA to plasmid DNA and back, allowing for the transfer and permanent addition of genes such as those encoding antibiotic resistance (multi-antibiotic resistant bacterial strains can be generated in this way). Bacterial transposons of this type belong to the Tn family. When the transposable elements lack additional genes, they are known as insertion sequences.
- The most common transposable element in humans is the Alu sequence. It is approximately 300 bases long and can be found between 300,000 and one million times in the human genome. Alu alone is estimated to make up 15–17% of the human genome.[20]
- Mariner-like elements are another prominent class of transposons found in multiple species, including humans. The Mariner transposon was first discovered by Jacobson and Hartl in Drosophila.[21] This Class II transposable element is known for its uncanny ability to be transmitted horizontally in many species.[22][23] There are an estimated 14,000 copies of Mariner in the human genome comprising 2.6 million base pairs.[24] The first mariner-element transposons outside of animals were found in Trichomonas vaginalis.[25] These characteristics of the Mariner transposon inspired the science fiction novel The Mariner Project by Bob Marr.
- Mu phage transposition is the best-known example of replicative transposition.
- Yeast (Saccharomyces cerevisiae) genomes contain five distinct retrotransposon families: Ty1, Ty2, Ty3, Ty4 and Ty5.[26]
- A helitron is a TE found in eukaryotes that is thought to replicate by a rolling-circle mechanism.
- Silk fiber is majorly composed of a fibrous protein called fibroin, which has hundreds of tandem repeat motifs derived through transposible elements during evolution, this confers tight packing and high crystallinity that offers tensile strength and lustrous nature to silk. In 2015, Adarsh Gupta K et al of Nagaraju's research team at Centre for DNA Fingerprinting and Diagnostics, Hyderabad, India discovered the protein structure of Silk Fibroin of Indian golden silkmoth, and published in Nature (journal) Scientific Reports [27]
In disease
TEs are mutagens and their movements are often the causes of genetic disease. They can damage the genome of their host cell in different ways:[28]
- a transposon or a retrotransposon that inserts itself into a functional gene will most likely disable that gene;
- after a DNA transposon leaves a gene, the resulting gap will probably not be repaired correctly;
- multiple copies of the same sequence, such as Alu sequences, can hinder precise chromosomal pairing during mitosis and meiosis, resulting in unequal crossovers, one of the main reasons for chromosome duplication.
Diseases often caused by TEs include hemophilia A and B, severe combined immunodeficiency, porphyria, predisposition to cancer, and Duchenne muscular dystrophy.[29][30] LINE1 (L1) TEs that land on the human Factor VIII have been shown to cause haemophilia[31] and insertion of L1 into the APC gene causes colon cancer, confirming that TEs play an important role in disease development.[32]
Additionally, many TEs contain promoters which drive transcription of their own transposase. These promoters can cause aberrant expression of linked genes, causing disease or mutant phenotypes.
Rate of transposition, induction and defense
One study estimated the rate of transposition of a particular retrotransposon, the Ty1 element in Saccharomyces cerevisiae. Using several assumptions, the rate of successful transposition event per single Ty1 element came out to be about once every few months to once every few years.[33] Some TEs contain heat-shock like promoters and their rate of transposition increases if the cell is subjected to stress,[34] thus increasing the mutation rate under these conditions, which might be beneficial to the cell.
Cells defend against the proliferation of TEs in a number of ways. These include piRNAs and siRNAs,[35] which silence TEs after they have been transcribed.
If organisms are mostly composed of TEs, one might assume that disease caused by misplaced TEs is very common, but in most cases TEs are silenced through epigenetic mechanisms like DNA methylation, chromatin remodeling and piRNA, such that little to no phenotypic effects nor movements of TEs occur as in some wild-type plant TEs. Certain mutated plants have been found to have defects in methylation-related enzymes (methyl transferase) which cause the transcription of TEs, thus affecting the phenotype.[4][36]
One hypothesis suggests that only approximately 100 LINE1 related sequences are active, despite their sequences making up 17% of the human genome. In human cells, silencing of LINE1 sequences is triggered by an RNA interference (RNAi) mechanism. Surprisingly, the RNAi sequences are derived from the 5' untranslated region (UTR) of the LINE1, a long terminal which repeats itself. Supposedly, the 5' LINE1 UTR that codes for the sense promoter for LINE1 transcription also encodes the antisense promoter for the miRNA that becomes the substrate for siRNA production. Inhibition of the RNAi silencing mechanism in this region showed an increase in LINE1 transcription.[4][37]
Evolution
TEs are found in most life forms, and the scientific community is still exploring their evolution and their effect on genome evolution. It is unclear whether TEs originated in the last universal common ancestor, arose independently multiple times, or arose once and then spread to other kingdoms by horizontal gene transfer.[38] While some TEs confer benefits on their hosts, most are regarded as selfish DNA parasites. In this way, they are similar to viruses. Various viruses and TEs also share features in their genome structures and biochemical abilities, leading to speculation that they share a common ancestor.
Because excessive TE activity can damage exons, many organisms have developed mechanisms to inhibit their activity. Bacteria may undergo high rates of gene deletion as part of a mechanism to remove TEs and viruses from their genomes, while eukaryotic organisms typically use RNA interference to inhibit TE activity. Nevertheless, some TEs generate large families often associated with speciation events. Evolution often deactivates DNA transposons, leaving them as introns (inactive gene sequences). In vertebrate animal cells, nearly all 100,000+ DNA transposons per genome have genes that encode inactive transposase polypeptides.[39] In humans, all Tc1-like transposons are inactive. The first synthetic transposon designed for use in vertebrate cells, the Sleeping Beauty transposon system, is a Tc1/mariner-like transposon. It exists in the human genome as an intron and was activated through reconstruction.[40]
Large quantities of TEs within genomes may still present evolutionary advantages, however. Interspersed repeats within genomes are created by transposition events accumulating over evolutionary time. Because interspersed repeats block gene conversion, they protect novel gene sequences from being overwritten by similar gene sequences and thereby facilitate the development of new genes. TEs may also have been co-opted by the vertebrate immune system as a means of producing antibody diversity. The V(D)J recombination system operates by a mechanism similar to that of some TEs.
TEs can contain many types of genes, including those conferring antibiotic resistance and ability to transpose to conjugative plasmids. Some TEs also contain integrons, genetic elements that can capture and express genes from other sources. These contain integrase, which can integrate gene cassettes. There are over 40 antibiotic resistance genes identified on cassettes, as well as virulence genes.
Transposons do not always excise their elements precisely, sometimes removing the adjacent base pairs; this phenomenon is called exon shuffling. Shuffling two unrelated exons can create a novel gene product or, more likely, an intron.[41]
Applications
The first TE was discovered in maize (Zea mays) and is named dissociator (Ds). Likewise, the first TE to be molecularly isolated was from a plant (snapdragon). Appropriately, TEs have been an especially useful tool in plant molecular biology. Researchers use them as a means of mutagenesis. In this context, a TE jumps into a gene and produces a mutation. The presence of such a TE provides a straightforward means of identifying the mutant allele relative to chemical mutagenesis methods.
Sometimes the insertion of a TE into a gene can disrupt that gene's function in a reversible manner, in a process called insertional mutagenesis; transposase-mediated excision of the DNA transposon restores gene function. This produces plants in which neighboring cells have different genotypes. This feature allows researchers to distinguish between genes that must be present inside of a cell in order to function (cell-autonomous) and genes that produce observable effects in cells other than those where the gene is expressed.
TEs are also a widely used tool for mutagenesis of most experimentally tractable organisms. The Sleeping Beauty transposon system has been used extensively as an insertional tag for identifying cancer genes.[42]
The Tc1/mariner-class of TEs Sleeping Beauty transposon system, awarded Molecule of the Year in 2009,[43] is active in mammalian cells and is being investigated for use in human gene therapy.[44][45][46]
TEs are used for the reconstruction of phylogenies by the means of presence/absence analyses.[47]
De novo repeat identification
De novo repeat identification is an initial scan of sequence data that seeks to find the repetitive regions of the genome, and to classify these repeats. Many computer programs exist to perform de novo repeat identification, all operating under the same general principles.[48] As short tandem repeats are generally 1–6 base pairs in length and are often consecutive, their identification is relatively simple.[49] Dispersed repetitive elements, on the other hand, are more challenging to identify, due to the fact that they are longer and have often acquired mutations. However, it is important to identify these repeats as they are often found to be transposable elements (TEs).[48]
De novo identification of transposons involves three steps: 1) find all repeats within the genome, 2) build a consensus of each family of sequences, and 3) classify these repeats. There are three groups of algorithms for the first step. One group is referred to as the k-mer approach, where a k-mer is a sequence of length k. In this approach, the genome is scanned for overrepresented k-mers; that is, k-mers that occur more often than is likely based on probability alone. The length k is determined by the type of transposon being searched for. The k-mer approach also allows mismatches, the number of which is determined by the analyst. Some k-mer approach programs use the k-mer as a base, and extend both ends of each repeated k-mer until there is no more similarity between them, indicating the ends of the repeats.[48] Another group of algorithms employs a method called sequence self-comparison. Sequence self-comparison programs use databases such as AB-BLAST to conduct an initial sequence alignment. As these programs find groups of elements that partially overlap, they are useful for finding highly diverged transposons, or transposons with only a small region copied into other parts of the genome.[50] Another group of algorithms follows the periodicity approach. These algorithms perform a Fourier transformation on the sequence data, identifying periodicities, regions that are repeated periodically, and are able to use peaks in the resultant spectrum to find candidate repetitive elements. This method works best for tandem repeats, but can be used for dispersed repeats as well. However, it is a slow process, making it an unlikely choice for genome scale analysis.[48]
The second step of de novo repeat identification involves building a consensus of each family of sequences. A consensus sequence is a sequence that is created based on the repeats that comprise a TE family. A base pair in a consensus is the one that occurred most often in the sequences being compared to make the consensus. For example, in a family of 50 repeats where 42 have a T base pair in the same position, the consensus sequence would have a T at this position as well, as the base pair is representative of the family as a whole at that particular position, and is most likely the base pair found in the family’s ancestor at that position.[48] Once a consensus sequence has been made for each family, it is then possible to move on to further analysis, such as TE classification and genome masking in order to quantify the overall TE content of the genome.
Adaptive TEs
Transposable elements have been recognized as good candidates for stimulating gene adaptation, through their ability to regulate the expression levels of nearby genes.[51] Combined with their “mobility,” transposable elements can be relocated adjacent to their targeted genes, and control the expression levels of the gene, dependent upon the circumstances.
The study conducted in 2008, High Rate of Recent Transposable Element–Induced Adaptation in Drosophila melanogaster, used D. melanogaster that had recently migrated from Africa to other parts of the world, as a basis for studying adaptations caused by transposable elements. Although most of the TEs were located on introns, the experiment showed the significant difference on gene expressions between the population in Africa and other parts of the world. The four TEs that caused the selective sweep were more prevalent in D. melanogaster from temperate climates, leading the researchers to conclude that the selective pressures of the climate prompted genetic adaptation.[52] From this experiment, it has been confirmed that adaptive TEs are prevalent in nature, by enabling organisms to adapt gene expression as a result of new selective pressures.
However, not all effects of adaptive TEs are beneficial to the population. In the research conducted in 2009, A Recent Adaptive Transposable Element Insertion Near Highly Conserved Developmental Loci in Drosophila melanogaster, a TE, inserted between Jheh 2 and Jheh 3, revealed a downgrade in the expression level of both of the genes. Down regulation of such genes has caused drosophila to exhibit extended developmental time and reduced egg to adult viability. Although this adaptation was observed in high frequency in all non-African populations, it was not fixed in any of them.[53] This is not hard to believe, since it is logical for a population to favor higher egg to adult viability, therefore trying to purge the trait caused by this specific TE adaptation.
At the same time, there have been several reports showing the advantageous adaptation caused by TEs. In the research done with silkworms, An Adaptive Transposable Element insertion in the Regulatory Region of the EO Gene in the Domesticated Silkworm, a TE insertion was observed in the cis-regulatory region of the EO gene, which regulates molting hormone 20E, and enhanced expression was recorded. While populations without the TE insert are often unable to effectively regulate hormone 20E under starvation conditions, those with the insert had a more stable development, which resulted in higher developmental uniformity.[54]
These three experiments all demonstrated different ways in which TE insertions can be advantageous or disadvantageous, through means of regulating the expression level of adjacent genes. The field of adaptive TE research is still under development and more findings can be expected in the future.
See also
- Insertion sequence
- Intragenomic conflict
- P element
- Tn3 Transposon
- Tn10
- Transposon tagging
- Signature tagged mutagenesis
- Evolution of sexual reproduction
Notes
- Kidwell, M.G. (2005). "Transposable elements". In T.R. Gregory (ed.). The Evolution of the Genome. San Diego: Elsevier. pp. 165–221. ISBN 0-123-01463-8.
- Craig NL, Craigie R, Gellert M, and Lambowitz AM (ed.) (2002). Mobile DNA II. Washington, DC: ASM Press. ISBN 978-1-555-81209-6.
{{cite book}}
:|author=
has generic name (help)CS1 maint: multiple names: authors list (link) - Lewin B (2000). Genes VII. Oxford University Press. ISBN 978-0-198-79276-5.
References
- ^ McClintock, Barbara (June 1950). "The origin and behavior of mutable loci in maize". Proc Natl Acad Sci U S A. 36 (6): 344–55. Bibcode:1950PNAS...36..344M. doi:10.1073/pnas.36.6.344. PMC 1063197. PMID 15430309.
- ^ Bucher E, Reinders J, Mirouze M (November 2012). "Epigenetic control of transposon transcription and mobility in Arabidopsis". Current Opinion in Plant Biology. 15 (5): 503–10. doi:10.1016/j.pbi.2012.08.006. PMID 22940592.
- ^ "'Junk' DNA Has Important Role, Researchers Find". Science Daily. 21 May 2009.
- ^ a b c Pray, Leslie A. (2008). "Transposons: The jumping genes". Nature Education. 1 (1): 204.
- ^ a b c d e McGrayne, Sharon Bertsch (1998). Nobel Prize Women in Science: Their Lives, Struggles, and Momentous Discoveries (2nd ed.). Carol Publishing. p. 165. ISBN 978-0-9702256-0-3.
{{cite book}}
: Invalid|ref=harv
(help) - ^ a b c McGrayne 1998, p. 166
- ^ McGrayne 1998, p. 167
- ^ McClintock B (November 1953). "Induction of Instability at Selected Loci in Maize". Genetics. 38 (6): 579–99. PMC 1209627. PMID 17247459.
- ^ Des Jardins, Julie (2010). The Madame Curie Complex: The Hidden History of Women in Science. Feminist Press at CUNY. p. 246. ISBN 978-1-55861-655-4.
- ^ Fedoroff, Nina; Botstein, David, eds. (1 January 1992). The Dynamic Genome: Barbara McClintock's Ideas in the Century of Genetics. Cold Spring Harbor Laboratory Press. p. 2. ISBN 978-0-87969-422-7.
- ^ SanMiguel P, Tikhonov A, Jin YK, et al. (November 1996). "Nested retrotransposons in the intergenic regions of the maize genome". Science. 274 (5288): 765–8. doi:10.1126/science.274.5288.765. PMID 8864112.
- ^ Mills, R.E., Bennett, E.A., Iskow, R.C. and Devine, S.E. (2007). "Which transposable elements are active in the human genome?". Trends in Genetics. 23 (4): 183–191. doi:10.1016/j.tig.2007.02.006.
{{cite journal}}
: CS1 maint: multiple names: authors list (link) - ^ Kapitonov, VV; et al. (May 2008). "A universal classification of eukaryotic transposable elements implemented in Repbase". Nature Reviews Genetics. 9 (5): 411–2. doi:10.1038/nrg2165-c1. PMID 18421312.
- ^ Madigan M, Martinko J (editors) (2006). Brock Biolog of Microorganisms (11th ed.). Prentice Hall. ISBN 0-13-144329-1.
{{cite book}}
:|author=
has generic name (help) - ^ Kazazian HH Jr; Moran JV (May 1998). "The impact of L1 retrotransposons on the human genome". Nature Genetics. 19 (1): 19–24. doi:10.1038/ng0598-19. PMID 9590283.
- ^ Schnable; et al. (November 2009). "The B73 maize genome: complexity, diversity, and dynamics". Science. 326 (5956): 1112–1115. Bibcode:2009Sci...326.1112S. doi:10.1126/science.1178534. PMID 19965430.
- ^ Spradling AC, Rubin GM (October 1982). "Transposition of cloned P elements into Drosophila germ line chromosomes". Science. 218 (4570): 341–347. Bibcode:1982Sci...218..341S. doi:10.1126/science.6289435. PMID 6289435.
- ^ Rubin GM, Spradling AC (October 1982). "Genetic transformation of Drosophila with transposable element vectors". Science. 218 (4570): 348–353. Bibcode:1982Sci...218..348R. doi:10.1126/science.6289436. PMID 6289436.
- ^ Cesari F (15 October 2007). "Milestones in Nature: Milestone 9: Transformers, Elements in Disguise". Nature. doi:10.1038/nrg2254.
- ^ Kazazian HH, Moran JV (May 1998). "The impact of L1 retrotransposons on the human genome". Nat. Genet. 19 (1): 19–24. doi:10.1038/ng0598-19. PMID 9590283.
- ^ Jacobson JW, Medhora MM, Hartl DL (November 1986). "Molecular structure of a somatically unstable transposable element in Drosophila". Proc. Natl. Acad. Sci. U.S.A. 83 (22): 8684–8. doi:10.1073/pnas.83.22.8684. PMC 386995. PMID 3022302.
- ^ Lohe AR, Moriyama EN, Lidholm DA, Hartl DL (January 1995). "Horizontal transmission, vertical inactivation, and stochastic loss of mariner-like transposable elements". Mol. Biol. Evol. 12 (1): 62–72. doi:10.1093/oxfordjournals.molbev.a040191. PMID 7877497.
- ^ Lampe DJ, Witherspoon DJ, Soto-Adames FN, Robertson HM (April 2003). "Recent horizontal transfer of mellifera subfamily mariner transposons into insect lineages representing four different orders shows that selection acts only during horizontal transfer". Mol. Biol. Evol. 20 (4): 554–62. doi:10.1093/molbev/msg069. PMID 12654937.
- ^ Mandal PK, Kazazian HH (October 2008). "SnapShot: Vertebrate transposons". Cell. 135 (1): 192–192.e1. doi:10.1016/j.cell.2008.09.028. PMID 18854165.
- ^ Carlton JM, Hirt RP, Silva JC, et al. (January 2007). "Draft genome sequence of the sexually transmitted pathogen Trichomonas vaginalis". Science. 315 (5809): 207–12. doi:10.1126/science.1132894. PMC 2080659. PMID 17218520.
- ^ Kim JM, Vanguri S, Boeke JD, Gabriel A, Voytas DF (May 1998). "Transposable elements and genome organization: a comprehensive survey of retrotransposons revealed by the complete Saccharomyces cerevisiae genome sequence". Genome Res. 8 (5): 464–78. doi:10.1101/gr.8.5.464. PMID 9582191.
- ^ "Molecular architecture of silk fibroin of Indian golden silkmoth, Antheraea assama". Scientific Reports. 5. 2015. doi:10.1038/srep12706.
{{cite journal}}
: Unknown parameter|authors=
ignored (help) - ^ Belancio VP, Hedges DJ, Deininger P (March 2008). "Mammalian non-LTR retrotransposons: for better or worse, in sickness and in health". Genome Res. 18 (3): 343–58. doi:10.1101/gr.5558208. PMID 18256243.
- ^ Kazazian H.H.; Goodier J.L. (2002). "LINE drive: retrotransposition and genome instability". Cell. 110 (3): 277–80. doi:10.1016/S0092-8674(02)00868-1. PMID 12176313.
- ^ Kapitonov V.V., Pavlicek, A., Jurka, J. (2006). "Anthology of Human Repetitive DNA". Encyclopedia of Molecular Cell Biology and Molecular Medicine. doi:10.1002/3527600906.mcb.200300166.
{{cite journal}}
: CS1 maint: multiple names: authors list (link) - ^ Kazazian, HH Jr; et al. (1988). "Haemophilia A resulting from de novo insertion of L1 sequences represents a novel mechanism for mutation in man". Nature. 332 (6160): 164–6. doi:10.1038/332164a0. PMID 2831458.
- ^ Miki, Y.; et al. (February 1992). "Disruption of the APC gene by a retrotransposal insertion of L1 sequence in colon cancer". Cancer Research. 52 (3): 643–5. PMID 1310068.
- ^ Paquin CE, Williamson VM (5 October 1984). "Temperature Effects on the Rate of Ty Transposition". Science. 226 (4670): 53–55. Bibcode:1984Sci...226...53P. doi:10.1126/science.226.4670.53. PMID 17815421.
- ^ Dennis J. Strand; John F. McDonald (1985). "Copia is transcriptionally responsive to environmental stress". Nucleic Acids Research. 13 (12): 4401–4410. doi:10.1093/nar/13.12.4401. PMC 321795. PMID 2409535.
- ^ Wei-Jen Chung; Katsutomo Okamura; Raquel Martin; Eric C. Lai (3 June 2008). "Endogenous RNA Interference Provides a Somatic Defense against Drosophila Transposons". Current Biology. 18 (11): 795–802. doi:10.1016/j.cub.2008.05.006. PMC 2812477. PMID 18501606.
- ^ Miura A, Yonebayashi S, Watanabe K, Toyama T, Shimada H, Kakutani T (May 2001). "Mobilization of transposons by a mutation abolishing full DNA methylation in Arabidopsis". Nature. 411 (6834): 212–4. doi:10.1038/35075612. PMID 11346800.
- ^ Yang N, Kazazian HH (September 2006). "L1 retrotransposition is suppressed by endogenously encoded small interfering RNAs in human cultured cells". Nat. Struct. Mol. Biol. 13 (9): 763–71. doi:10.1038/nsmb1141. PMID 16936727.
- ^ Kidwell, M.G. (1992). "Horizontal transfer of P elements and other short inverted repeat transposons". Genetica. 86 (1): 275–286. doi:10.1007/BF00133726. PMID 1334912.
- ^ Plasterk R.H.A.; Izsvák Z.; Ivics Z. (1999). "Resident aliens: the Tc1/mariner superfamily of transposable elements". Trends Genet. 15 (8): 326–32. doi:10.1016/S0168-9525(99)01777-1. PMID 10431195.
- ^ Ivics Z.; Hackett P.B.; Plasterk R.H.; Izsvak Z. (1997). "Molecular reconstruction of Sleeping Beauty, a Tc1-like transposon from fish, and its transposition in human cells". Cell. 91 (4): 501–10. doi:10.1016/S0092-8674(00)80436-5. PMID 9390559.
- ^ Moran JV, DeBerardinis RJ, Kazazian HH (March 1999). "Exon shuffling by L1 retrotransposition". Science. 283 (5407): 1530–4. doi:10.1126/science.283.5407.1530. PMID 10066175.
- ^ Carlson C.M.; Largaespada D.A. (2005). "Insertional mutagenesis in mice: new perspectives and tools". Nature Reviews Genetics. 6 (7): 568–80. doi:10.1038/nrg1638. PMID 15995698.
- ^ Luft FC (May 2010). "Sleeping Beauty jumps to new heights". Mol. Med. 88 (7): 641–643. doi:10.1007/s00109-010-0626-1. PMID 20467721.
- ^ Ivics Z, Izsvák Z (October 2006). "Transposons for gene therapy!". Curr Gene Ther. 6 (5): 593–607. doi:10.2174/156652306778520647. PMID 17073604.
- ^ Wilson MH, Coates CJ, George AL (January 2007). "PiggyBac transposon-mediated gene transfer in human cells". Mol. Ther. 15 (1): 139–145. doi:10.1038/sj.mt.6300028. PMID 17164785.
- ^ Hackett P.B.; Largaespada D.A.; Cooper L.J.N. (2010). "A transposon and transposase system for human application". Mol. Ther. 18 (4): 674–83. doi:10.1038/mt.2010.2. PMC 2862530. PMID 20104209.
- ^ Jan Ole Kriegs, Gennady Churakov, Martin Kiefmann, Ursula Jordan, Jürgen Brosius, Jürgen Schmitz, David Penny: Retroposed Elements as Archives for the Evolutionary History of Placental Mammals. In: PLoS Biology. 4, 2006, S. e91, doi:10.1371/journal.pbio.0040091.
- ^ a b c d e Makałowski W, Pande A, Gotea V, Makałowska I (2012). "Transposable elements and their identification". Methods Mol. Biol. 855: 337–59. doi:10.1007/978-1-61779-582-4_12. PMID 22407715.
- ^ Saha S, Bridges S, Magbanua ZV, Peterson DG (2008). "Computational Approaches and Tools Used in Identification of Dispersed Repetitive DNA Sequences". Tropical Plant Biol. 1: 85–96. doi:10.1007/s12042-007-9007-5.
- ^ Saha S, Bridges S, Magbanua ZV, Peterson DG (April 2008). "Empirical comparison of ab initio repeat finding programs". Nucleic Acids Res. 36 (7): 2284–94. doi:10.1093/nar/gkn064. PMC 2367713. PMID 18287116.
- ^ Mariño-Ramírez, L., Lewis, K. C., Landsman, D., & Jordan, I. K. (2005). "Transposable elements donate lineage-specific regulatory sequences to host genomes". Cytogenetic and Genome Research. 110 (1–4): 333–41. doi:10.1159/000084965.
{{cite journal}}
: CS1 maint: multiple names: authors list (link) - ^ González, J., Lenkov, K., Lipatov, M., Macpherson, J. M., & Petrov, D. A. (2008). "High Rate of Recent Transposable Element–Induced Adaptation in Drosophila melanogaster". PLoS Biology. 6 (10): e251. doi:10.1371/journal.pbio.0060251.
{{cite journal}}
: CS1 maint: multiple names: authors list (link) CS1 maint: unflagged free DOI (link) - ^ González, J., Macpherson, J. M., & Petrov, D. A. (2009). "A Recent Adaptive Transposable Element Insertion Near Highly Conserved Developmental Loci in Drosophila melanogaster". Molecular Biology and Evolution. 26 (9): 1949–1961. doi:10.1093/molbev/msp107.
{{cite journal}}
: CS1 maint: multiple names: authors list (link) - ^ Sun, W., Shen, Y., Han, M., Cao, Y., & Zhang, Z. (2014). "An Adaptive Transposable Element insertion in the Regulatory Region of the EO Gene in the Domesticated Silkworm, Bombyx mori". Molecular Biology and Evolution. 31 (12): 3302–3313. doi:10.1093/molbev/msu261.
{{cite journal}}
: CS1 maint: multiple names: authors list (link)
External links
- "An immune system so versatile it might kill you". New Scientist (2556). 21 June 2006. — A possible connection between aberrant reinsertions and lymphoma.
- Repbase — a database of transposable element sequences
- RepeatMasker — a computer program used by computational biologists to annotate transposons in DNA sequences
- Use of the Sleeping Beauty Transposon System for Stable Gene Expression in Mouse Embryonic Stem Cells