Steam locomotive
British Railways Standard Class 9F 2-10-0 no. 92214 (preserved) at Barrow Hill, October 10, 2004

A steam locomotive is a locomotive powered by steam. The term usually refers to its use on railways, but can also refer to a "road locomotive" such as a traction engine or steamroller.
Steam locomotives dominated rail traction from the mid 19th century until the mid 20th century, after which they were superseded by diesel and electric locomotives.
Many consider the steam locomotive to be one of the most fascinating mechanical devices ever created and passing steam locomotives often grab the attention of bystanders.
Origins
The earliest railways employed horses to draw carts over the track. As steam engines were developed in the 1700s, various attempts were made to apply these to road and railroad use.[1] The first attempts were made in Great Britain; the earliest steam locomotive was built in 1804 by Richard Trevithick and Andrew Vivian. It ran with mixed success on the narrow gauge "Penydarren" (Merthyr Tydfil) tramway in Wales.[1]. Then followed the successful twin cylinder locomotives built by Matthew Murray for the edge railed Middleton Railway in 1812. These early efforts culminated in 1829 with Stephenson's Rocket, which was the first viable[citation needed] mainline locomotive.[2]

Inspired by British success, the United States started developing steam locomotives in 1829 with the Baltimore and Ohio Railroad's Tom Thumb. This was the first locomotive to run in America, although it was intended as a demonstration of the potential of steam traction, rather than as a revenue-earning locomotive. The first successful steam railway in the US was the South Carolina Railroad whose inaugural train ran in December 1830 hauled by the Best Friend of Charleston. Many of the earliest locomotives for American railroads were imported from England, including the Stourbridge Lion and the John Bull, but a national locomotive manufacturing industry was quickly established, with locomotives like the DeWitt Clinton being built in the 1830s.[2]
See also: History of rail transport, Category:Early steam locomotives
Basic form

Boiler
The typical steam locomotive employs a horizontal fire-tube boiler with the firebox at the rear. The boiler projects slightly into a cab, which shields the locomotive operators from the weather. At the front of the boiler is the smokebox, with chimney (US: "smoke stack") protruding from the top. Steam is collected from the top of the boiler, either in a perforated tube fitted above the water level or from a dome. This system reduces the risk of water entering the cylinders.
Cylinders
The steam passes through a throttle (known as the "regulator valve"), then into the cylinders of a reciprocating engine. The pistons drive the driving wheels directly through a connecting rod (US: "main rod") and a crankpin on the main driver. The valves of the engine are controlled through a set of rods and linkages called the valve gear; this gear is adjustable to control the direction and cut-off of the valve gear. The cut-off point determines the proportion of the stroke, during which steam is admitted into the cylinder; for example a 50% cut-off admits steam for half the stroke of the piston. The remainder of the stroke is driven by the expansive force of the steam. Careful use of cut-off provides economical use of steam and, in turn, reduces fuel and water consumption. The reversing lever (US: "Johnson bar", or "screw-reverser" if so equipped) which controls the cut-off therefore performs a similar function to a gearshift in an automobile. Exhaust steam escapes the engine through the chimney, via a nozzle called a blastpipe. This action creates a draft through the fire grate according to the steam exhausted. The blast of exhausted steam produces the familiar "chuffing" sound of the steam locomotive.
The pistons are double acting, with steam admitted to either end in turn. In a two-cylinder locomotive, one cylinder is located on either side of the locomotive. The cylinders are set 90 degrees out of phase with each other. During a full rotation of the driving wheel, steam provides four power strokes per revolution; that is to say each cylinder receives two injections of steam. The first stroke is to the front of the piston and the second stroke to the rear of the piston; hence two working strokes. Each steam stroke delivers a quarter turn of the wheel. Consequently two deliveries of steam onto each piston face in two cylinders generates a full revolution of the driving wheel. The driving wheels are connected on each side by coupling rods (US: "connecting rods") to transmit power from the main driver to the other wheels. At the two "dead centres", when the connecting rod is on the same axis as the crankpin on the driving wheel, it will be noted that no turning force can be applied. If the locomotive were to come to rest in this position it would be impossible for it to move off again, so the cylinders and crankpins are arranged such that the dead centres occur out of phase with each other. This precaution is unnecessary on most other reciprocating engines (such as an internal combustion engine) which are never expected to start from rest under their own power, and employ a flywheel to overcome the dead centres.
Frame
The boiler rests on a frame, to which the cylinders are mounted and which in turn rest on the axles. The driving axles are mounted in bearings which move up and down in the frame. They are also connected to the frame via leaf springs or, less commonly, volute springs and linkages which allow axles some degree of independent movement over bumps in the track. Many locomotives have leading bogies (US: "trucks") to guide them into curves and/or a trailing bogie to support the firebox weight.
British locomotives usually have plate frames (made from steel plate) while American locomotives usually have bar frames (made from steel bar) or cast steel frames. Each method has significant advantages and disadvantages.
Fuel and water
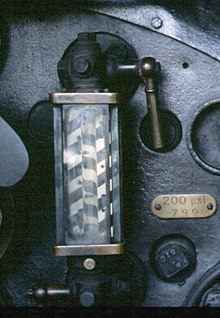
Generally, the largest locomotives are permanently coupled to a tender that carries the water and fuel. Alternatively, smaller locomotives carry the fuel in a bunker attached to the cab, and the water in tanks mounted on the engine, either beside the boiler or on top; these are called "tank engines".
The fuel source used depends on what is economically available locally to the railway. In the UK and parts of Europe, a plentiful supply of coal made this the obvious choice from the earliest days of the steam engine. Wood-burning engines were found in rural and logging districts in Europe and in the U.S.A. well into the 19th century. Bagasse, a waste by-product of the refining process, was burned in sugar cane farming operations. In the USA, the ready availability of oil made this a popular steam locomotive fuel; the Southern Pacific, for example, went directly from wood to oil.[citation needed] In Victoria, Australia after World War II, many steam locomotives were converted to oil firing. Also in Australia, waste oil fired the West Cost Rail's regular 4-6-4 steam passenger service during the late 1990s[citation needed]. German, Russian and Australian railways experimented using coal dust to fire locomotives.
Water was supplied at passenger stations and loco depots from a dedicated water tower connected to water cranes or gantries. In the UK and the USA, water troughs (track pans) were provided on some main lines to allow express trains to replenish their water supply without stopping. This was achieved by using a 'water scoop' fitted under the tender; the fireman remotely lowered the scoop into the trough, the speed of the engine forced the water into the tender, and the scoop was raised again once the tank was full.
Water is an essential element in the operation of a steam locomotive; because as Swengel argued, 'it has the highest specific heat of any common substance; that is more thermal energy is stored by heating water to a given temperature than would be stored by heating an equal mass of steel or copper to the same temperature. In addition, the property of vapourising (forming steam) stores additional energy without increasing the temperature...water is avery satisfactory medium for converting thermal energy of fuel into mechanical energy.'
Said Swengel, 'at low temperature and relatively low boiler outputs' good water and regular boiler washout was an acceptable practise, even though such maintenance was high. As steam pressures increased, however, a problem of 'foaming' or 'priming' developed, wherein dissolved solids in the water formed 'tough skined bubbles' inside the boiler, which inturn were carried into the steam pipes and blew off the cylinder heads. To overcome the problem, hot mineral concentrated water was deliberately wasted (blowing down)from the boiler from time to time. Higher steam pressures required more blowing down of water out of the boiler. Oxygen generated by boiling water attacks the boiler and with increased steam pressures the rate of rust (iron oxide) generated inside the boiler increases. One way to help overcome the problem was water treatment. Swengel suggested that the problems around water, contributed to the interest in electrification of railways. [3]
Crew
A crew of at least two people is required to operate a steam locomotive. One, the driver or engineer, is responsible for controlling the locomotive and the other, the boilerman or fireman, is responsible for the fire, steam pressure, and water.[4]
See also Category:locomotive parts
Fittings and appliances
All locomotives are fitted with a variety of appliances. Some of these relate directly to the operation of the steam engine; while others are for signalling, train control, or other purposes. In the United States the Federal Railroad Administration mandated the use of certain appliances over the years in response to safety issues. The most typical appliances are as follows:
Steam pumps and injectors
Water must be forced into the boiler, to replace that which is exhausted after delivering a working stroke to the pistons. Early engines used pumps driven by the motion of the pistons. Later steam injectors replaced the pump, while some engines use turbopumps. Standard practice evolved to use two independent systems for feeding water to the boiler. Vertical glass tubes, known as water gauges or water glasses, show the level of water in the boiler.
Boiler lagging
Large amounts of heat are wasted if a boiler is not insulated. Early locomotives used shaped wooden battens fitted lengthways along the boiler barrel and held in place by metal bands. Improved insulating methods included: applying a thick paste containing a porous mineral, such as kieselgur; fixing "mattresses" of stitched asbestos cloth stuffed with asbestos fibre around (but on separators so as not quite to touch) the boiler; attaching shaped blocks of insulating compound. The most common modern day material is glass wool, or wrappings of aluminium foil. The installation is covered by a close fitted sheet-metal casing.[5] Effective lagging is particularly important for fireless locomotives.
Pressure gauge

The earliest locomotives did not show the pressure of steam in the boiler, but it was possible to estimate this by the state of the safety valve. However, the promoters of the Rainhill trials urged that each contender have a proper mechanism for reading the boiler pressure and Stephenson devised a nine-foot vertical glass tube of mercury, mounted alongside the chimney, for the Rocket. The Bourdon tube gauge, in which the pressure straightens an oval-section, coiled tube of brass or bronze connected to a pointer, was not introduced until the 1850s. This is the device used today.[6] Some locomotives have an additional pressure gauge in the steam chest. This helps the driver avoid wheel-slip at startup, by warning if the regulator opening is too great.
Superheating
In the 19th century most engines used saturated steam. Post-1900 superheated steam appeared in the U.S.A. Prior to superheating locomotive development had almost reached its practical capacity with saturated steam. Swengel (1967:122) said that 'no single development ever equalled the superheater as a means of removing limitations from steam locomotive design'.
The normal method for superheating is to route the steam from the dome to a header in the smokebox. The steam is then directed through a set of small "U" shaped tubes in enlarged boiler tubes, and then into a second header and on through the cylinders. Superheating produces an increase in efficiency of 10-15% for an increase in temperature of 100 to 150 F° (55 to 85 C°). Although some locomotives have a greater degree of superheat applied, higher temperatures do not offer proportionally greater efficiencies. Superheated locomotives were sometimes fitted with pyrometers to indicate the steam temperature which, towards the end of the steam era, was typically around 600°F (315°C).
One pound of saturated steam at 200 p.s.i. occupies 2.134 cubic feet of space and when admitted to the cylinders the water (condensation) content is about 7%. At higher boiler pressure the water content in the cylinder increases. Prior to superheating, the boiler limit for any given locomotive was 200 p.s.i. as a locomotive could not as a rule, handle more than 7% water in its cylinders. Superheating, in short, dries out the steam, increases its volume and this gain increases the amount of work delivered from the same one pound of water. For this reason, it was common to find that the best improvements did not occur until locomotives were some way into their overhaul cycle and leaks had started to develop.[7] The use of superheaters became standard in all high-powered locomotives of the 20th century.
Superheating increased the tractive effort of a modified locomotive over its prior saturated condition. A bad practice which followed the initial introduction of superheating involved dropping the boiler pressure to achieve the same tractive effort, increasing the cylinder size to obtain increased work and removing many small tubes and replacing them with larger diameter flues to hold the superheaters. These modifications actually lessened the amount of evaporate surface, compared with the old saturated boiler, and limited the capacity of the locomotive.[8] Had the boiler pressure remained the same following superheating the overall effectiveness of the locomotive would have increased. By the mid-1920s, designers understood that superheating, large furnaces and higher boiler capacity were the key to successful locomotives.[8]
Stokers
A factor that limits locomotive performance is the rate at which fuel is fed into the fire. In the early 20th century some locomotives became so large, that the fireman could not shovel coal fast enough.[citation needed] In the United States, various steam-powered mechanical stokers became standard equipment and were adopted and used elsewhere including Australia and South Africa.
Feedwater heaters
Introducing cold water into a boiler reduces power, and from the 1920s a variety of heaters extracted waste heat from the exhaust and raised the temperature of the feed water. An example of the pre-heater is found on the Franco-Crosti boiler.
The use of live steam and exhaust steam injectors also assists in the pre-heating of boiler feed water, though there is no efficiency advantage to live steam injectors. Such pre-heating also reduces the thermal shock that a boiler might experience when cold water is introduced directly.
Condensers and water re-supply
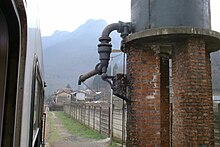
Steam locomotives consume vast quantities of water, and supplying this was a constant logistical problem. In some desert areas, condensing engines were devised. These engines had huge radiators in their tenders and instead of exhausting steam out of the funnel it was captured and passed back to the tender and condensed. The cylinder lubricating oil was removed from the exhausted steam to avoid a phenomenon known as priming, a condition caused by foaming in the boiler which would allow water to be carried into the cylinders causing damage because of its incompressibility. The most notable engines employing condensers (Class 25C) worked across the Karoo desert of South Africa, from the 1950 until the 1980s.
Some British and American locomotives were equipped with scoops which collected water from "water troughs" (US: "track pans") while in motion, thus avoiding stops for water. In the US, small communities that were established near sections of the railroad with track pans were often referred to as "jerkwater towns" (a term which today is considered derisive). In Australia and South Africa, locomotives in drier regions operated with large oversized tenders and some even had an additional water wagon, sometimes called a "canteen".
Steam locomotives working on underground railways (such as London's Metropolitan Railway) were fitted with condensing apparatus for a different, but obvious, reason. These were still being used between King's Cross and Moorgate into the 1950s.
Braking
Locomotives have their own braking system, independent from the rest of the train. Locomotive brakes employ large shoes which press against the driving wheel treads. With the advent of air brakes, a separate system also allowed the driver to control the brakes on all cars. These systems require steam-powered pumps, which are mounted on the side of the boiler or on the smokebox front. Such systems operated in the United States, Canada and Australia.
An alternative to the air brake is the vacuum brake. Where vacuum brakes are used, a steam-operated ejector is mounted on the engine instead of the air pump. A secondary ejector or crosshead vacuum pump is used to maintain the vacuum in the system. Vacuum systems existed on British, Indian and South African rail networks.
Steam locomotives are nearly always fitted with sandboxes from which sand can be delivered to the rails to improve traction and braking in wet weather. On American locomotives the sandboxes, or sand domes, are usually mounted on top of the boiler. In Britain, the limited loading gauge precludes this, so the sandboxes are mounted just above, or just below, the running plate.
Lubrication
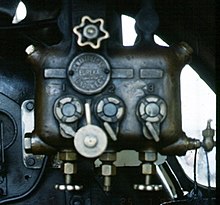
The pistons and valves on the earliest locomotives were lubricated by the enginemen dropping a lump of tallow down the blast pipe.[9]
As speeds and distances increased, mechanisms were developed that injected thick mineral oil into the steam supply. The first, a displacement lubricator, mounted in the cab, uses a controlled stream of steam condensing into a sealed container of oil. Water from the condensed steam displaces the oil into pipes. The apparatus is usually fitted with sight-glasses to confirm the rate of supply. A later method used a mechanical pump worked from one of the crossheads. In both cases, the supply of oil is proportional to the speed of the locomotive.

Lubricating the frame components (axle bearings, horn blocks and bogie pivots) depends on capillary action: trimmings of worsted yarn are trailed from oil reservoirs into pipes leading to the respective component.[10] The rate of oil supplied is controlled by the size of the bundle of yarn and not the speed of the locomotive, so it is necessary to remove the trimmings (which are mounted on wire) when stationary. However, at regular stops (such as a terminating station platform) oil finding its way onto the track can still be a problem.
Crank pin and crosshead bearings carry small cup-shaped reservoirs for oil. These have feed pipes to the bearing surface that start above the normal fill level, or are kept closed by a loose-fitting pin, so that only when the locomotive is in motion does oil enter. In United Kingdom practice the cups are closed with simple corks, but these have a piece of porous cane pushed through them to admit air. It is customary for a small capsule of pungent oil (aniseed or garlic) to be incorporated in the bearing metal to warn if the lubrication fails and excess heating or wear occurs.
Buffers
In British practice, the locomotive usually had buffers at each end to absorb compressive loads ("buffets"[11]). The tensional load of drawing the train (draft force) is carried by the coupling system. Together these control slack between the locomotive and train, absorb minor impacts, and provide a bearing point for pushing movements.
In American practice all of the forces between the locomotive and cars are handled through the coupler and its associated draft gear, which allows some limited slack movement. Small dimples called "poling pockets" at the front and rear corners of the locomotive allowed wagons to be pushed on an adjacent track using a pole braced between the locomotive and the cars.
Pilots
In the United States, South Africa and Australia, locomotives had a pilot at the front end. Plow-shaped, and called cow catchers, they were quite large and were designed to remove obstacles from the track such as cattle, bison, other animals or tree limbs. Though unable to "catch" stray cattle these distinctive items remained on locomotives in those countries until the end of steam. Switching engines usually replaced the pilot with small steps. In places like Victoria, Australia, the cow catchers became a trade mark of that state's engines (Dee:1998).
Headlights

When night operations began, railway companies in some countries equipped their locomotives with lights to allow the driver to see what lay ahead of the train or to enable others to see the locomotive. Originally headlights were oil or acetylene lamps, but when electric lights became available in the late 1880s, they quickly replaced the older types.
Britain used low intensity oil lamps and were not intended to allow the driver to see the way ahead (locomotive drivers were expected to have sufficient route knowledge) but were used to indicate the class of a train by their position on the front of the locomotive. Four lamp irons were provided: one below the chimney and three evenly spaced across the top of the buffer beam.
In some countries heritage steam operation continues on the national network. Some railway authorities have mandated powerful headlights on at all times, including during daylight. This was to further inform the public or track workers of any active trains.
Bells and whistles
Locomotives used bells and steam whistles from earliest days. In the United States and Canada bells warned of a train in motion. In Britain, where all lines are by law fenced throughout,[12] bells were never a requirement; whistles are used to signal personnel and give warnings. In Australia, locomotives carried distinctive whistles heard at long distances.
Automatic Train Control
From early in the twentieth century operating companies in such countries as Germany and Britain began to fit locomotives with in-cab signalling which automatically applied the brakes when a signal was passed at "caution". In Britain these became mandatory in 1956.
Booster engines
In the United States and Australia the trailing truck was often equipped with an auxiliary steam engine which provided extra power for starting. This booster engine was set to cut out automatically at a certain speed. On the narrow gauged New Zealand railway system, six Kb 4-8-4 locomotives had boosters; the only (3ft 6 in) metre engines in the world to have such equipment.
Variations
Numerous variations to the simple locomotive occurred as railways attempted to develop more powerful, more efficient and fast steam locomotives.
Cylinders
Some locomotives received extra cylinders and experiments combined two locomotives in one (e.g. the Mallet and Garratt locomotives). Some locomotives carried their cylinders vertically alongside the boiler and drove the wheels through a system of shafts and gears (e.g. the Shay locomotive; see "geared steam locomotive").
From about 1930, most new British express passenger locomotives were 4-6-0 or 4-6-2 types with three or four cylinders. Examples include:
- GWR 4073 Class, four-cylinder 4-6-0
- LNER Class A1/A3, three-cylinder 4-6-2
Cab forward
In the United States on the Southern Pacific Railroad a series of cab forward locomotives had the cab and the firebox at the front of the locomotive and the tender behind the smokebox, so that the engine appeared to run backwards. This was only possible by using oil-firing. Another variation was the Camelback type locomotive with the cab half-way along the boiler.
Steam turbines
Experiments with steam turbines using direct-drive and electrical transmissions, in different countries, proved unsuccessful.[5] The LMS also built Turbomotive, an attempt to prove the efficiency of steam turbines.[5] In the United States the Union Pacific, Chesapeake and Ohio, and Norfolk & Western railways all built turbine-electric locomotives. The Pennsylvania Railroad (PRR) also built turbine locos but with a direct-drive gearbox. However, all designs failed due to dust, vibration, design flaws, or inefficiency below speed. The last one in service was the N&W's being retired in January 1958. The longest in service was the PRR's with 6 years of service (1944-1950).
Valve gear
Numerous technological advances improved the steam engine. Early locomotives used simple valve gear that gave full power in either forward or reverse.[6] Soon Stephenson valve gear allowed the driver to control cutoff; this was largely superseded by Walschaerts valve gear and similar patterns. Early locomotive practices of slide valves and outside admission, which were easy to construct but inefficient and prone to wear.[6] Eventually, slide valves were superseded by inside admission piston valves, though there were attempts to apply poppet valves (common by then on stationary engines) in the 20th century. Stephenson valve gear was generally placed within the frame and was difficult to access for maintenance; later patterns applied outside the frame, were readily visible and maintained.
Compounding
Around 1900, compound locomotives appeared, which used the engine's steam twice. Some attempts were made to apply this to a single engine (e.g. the Vauclain compound) but the predominant form was the Mallet locomotive, which used two separate engines in one articulated frame. The high pressure stage was attached directly to the boiler frame; in front of this was a separate low pressure engine on its own frame, powered by the exhaust from the rear engine. Other prototypes included the LMS a parallel high-low pressure boilered locomotive, called Fury.
Articulated and Duplex types
Articulation itself proved very popular, and there were numerous variations, both compound and simple. Duplex locomotives with two engines in one rigid frame were also tried, but were not notably successful.[citation needed]
Hybrid power
Mixed power locomotive prototypes have been produced. The LNER had a prototype Kitson-Still hybrid built for testing, using a steam and diesel engine on a 2-6-2 wheelbase. It proved more efficient, but its running costs were 25% higher than an equivalent steam locomotive.
Manufacture
United States
With the notable exception of the USRA standard locomotives, set during World War 1, in the United States, steam locomotive manufacture was always customised. Railroads ordered locomotives tailored to their specific requirements, though basic similarities were always present. Railroads developed specific characteristics; for example, the Pennsylvania Railroad had a preference for the Belpaire firebox,[citation needed] while the Delaware and Hudson Railroad was famous for its elaborately flanged smokestacks.[citation needed] In the United States, specialised manufacturers constructed locomotives for all rail companies, although all railroads had shops capable of heavy repairs and some railroads(for example the Norfolk and Western Railway) constructed locomotives in their own shops. It was not uncommon for a group of locomotives to be sold from one railroad to another.[citation needed]
Steam locomotives required regular service and overhaul (often at government-regulated intervals). Many alterations occurred during overhauls. New appliances were added, unsatisfactory features removed, cylinders improved or replaced. Any part of the locomotive, including boilers were replaced or upgraded. On the Baltimore and Ohio Railroad two 2-10-2 locomotives were dismantled; the boilers were placed onto two new Class T 4-8-2 locomotives and the residue wheel machinery made a pair of Class U 0-10-0 switchers with new boilers. Union Pacific's fleet of 3 cylinder 4-10-2 engines were converted into two cylinder engines in 1942, because of high maintenance problems.

United Kingdom
Before the 1923 Grouping, the picture in the UK was mixed. The larger railway companies built locomotives in their own workshops but the smaller ones and industrial concerns ordered them from outside builders.
Between 1923 and 1947, the "Big Four" railway companies (the Great Western Railway, the London, Midland and Scottish Railway, the London and North Eastern Railway and the Southern Railway) all built most of their own locomotives. Generally speaking, they only bought locomotives from outside builders when their own works were fully occupied. From 1948, British Railways adopted the same policy and continued to build new steam locomotives until 1960 (the last being named Evening Star).
Some independent manufacturers produced steam locomotives for a few more years, the last British-built industrial steam locomotive being constructed by Hunslet in 1971. Since then, a few specialised manufacturers have continued to produce small locomotives for narrow gauge and miniature railways, but as the prime market for these is the tourist and heritage railway sector, the demand for such locomotives is limited.
Australia
In Australia, Clyde Engineering of Sydney and also the Eveleigh Workshops built steam locomotives for the New South Wales Government Railways. These include the C38 class 4-6-2, the first five were build at Clyde with streamlining, the other 25 locomotives were built at Eveleigh (13) in Sydney, and Cardiff Workshops (12) near Newcastle. In Queensland, steam locomotives were locally constructed by Walkers. Similarly the South Australian state government railways also manufactured steam locomotives locally at Islington in Adelaide. The Victorian Railways constructed most of their locomotives at their Newport Workshops and Bendigo while in the early days locomotives were built in Ballarat. Locomotives constructed at the Newport shops ranged from the nA class 2-6-2T built for the narrow gauge, up to the H class 4-8-4, the largest conventional locomotive ever to operate in Australia, which weighed 260 tons. However, the title of largest locomotive in Australia goes to the 263 ton NSWGR AD60 class 4-8-4+4-8-4 Garratt (Oberg:1975), which were built by Beyer-Peacock in the United Kingdom.
Categorisation

Steam locomotives are categorised by their wheel arrangement. The two dominant systems for this are the Whyte notation and UIC classification.
The Whyte notation, used in most English speaking and Commonwealth countries, represents each set of wheels with a number. Different arrangements were given names which usually reflect the first usage of the arrangement; for instance the "Santa Fe" type (2-10-2) is so called because the first examples were built for the Atchison, Topeka and Santa Fe Railroad. These names were informally given and varied according to region and even politics.
The UIC classification is used mostly in European countries apart from the United Kingdom. It designates consecutive pairs of wheels (informally "axles") with a number for non-driving wheels and a capital letter for driving wheels (A=1, B=2 etc). So a Whyte 4-6-2 designation would be an equivalent to a 2-C-1 UIC designation.
On many railroads, locomotives were organised into classes. These broadly represented locomotives which could be substituted for each other in service, but most commonly a class represented a single design. As a rule classes were assigned some sort of code, generally based on the wheel arrangement. Classes also commonly acquired nicknames representing notable (and sometimes uncomplimentary) features of the locomotives.[citation needed]
Performance
Measurement
In the steam locomotive era two measures of locomotive performance were generally applied. At first, locomotives were rated by tractive effort This can be roughly calculated by multiplying the total piston area by 85% of the boiler pressure (a rule of thumb reflecting the slightly lower pressure in the steam chest above the cylinder) and dividing by the ratio of the driver diameter over the piston stroke.[7] However, the precise formula is:
Tractive Effort is defined as the average force developed during one revolution of the driving wheels at the rail head.[8] This is expressed as:
T.E. = (B² x S x 0.85P) ÷ D
B is bore of cylinder (diameter)in inches. S is cylinder stroke, in inches. P is boiler pressure in pound per square inch; and D is driving wheel diameter in inches
It is critical to appreciate the use of the term 'average', as not all effort is constant during the one revolution of the drivers for at some points of the cycle only one piston is exerting turning moment and at other points both pistons are working. Not all boilers deliver full power at starting and also the tractive effort decreases as the rotating speed increases.[8]
Tractive effort is a measure of the heaviest load a locomotive can start or haul at very low speed over the ruling grade in a given territory.[8]
However, as the pressure grew to run faster freight and heavier passenger trains, tractive effort was seen to be an inadequate measure of performance because it did not take into account speed.
Therefore in the 20th century, locomotives began to be rated by power output. A variety of calculations and formulas were applied, but in general railroads used dynamometer cars to measure tractive force at speed in actual road testing. This measure was termed drawbar horsepower in the United States and remained the standard measure of performance to the end of mainline usage.[citation needed]
British railway companies have been reluctant to disclose figures for drawbar horsepower and have usually relied on continuous tractive effort instead.
Relation to wheel arrangement
Whyte classification is connected to locomotive performance, but through a somewhat circuitous path. Given adequate proportions of the rest of the locomotive, power output is determined by the size of the fire, and for a bituminous coal-fuelled locomotive, this is determined by the grate area. Modern non-compound locomotives are typically able to produce about 40 drawbar horsepower per square foot of grate. Tractive force, as noted earlier, is largely determined by the boiler pressure, the cylinder proportions, and the size of the drivers. However, it is also limited by the weight on the drivers (termed adhesive weight), which needs to be at least four times the tractive effort.[5]
The weight of the locomotive is roughly proportional to the power output; the number of axles required is determined by this weight divided by the axleload limit for the trackage where the locomotive is to be used. The number of drivers is derived from the adhesive weight in the same manner, leaving the remaining axles to be accounted for by the leading and trailing bogies.[5] Passenger locomotives conventionally had two-axle leading bogies for better guidance at speed; on the other hand, the vast increase of the grate and firebox in the 20th century meant that trailing bogie was called upon to provide its support.
As a rule, "shunting engines" (US "switching engines") omitted leading and trailing bogies, both to maximise tractive effort available and to reduce wheelbase. Speed was unimportant; making the smallest engine (and therefore smallest fuel consumption) for the tractive effort paramount. Drivers were small and usually supported the firebox as well as the main section of the boiler. Helper engines tended to follow the principles of switchers, except that the wheelbase limitation did not apply. Therefore helpers tended to multiply the number of drivers, leading eventually to the Mallet type with its many driven wheels. These tended to acquire leading and then trailing bogies as guidance of the engine became more of an issue.
As locomotive types began to diverge in the late 1800s, freight engines at first emphasised tractive effort, whereas passenger engines emphasised speed. Freight locomotives multiplied axles, kept the leading bogie to a single axle, and grew a trailing bogie as the firebox expanded and could no longer fit between or above the drivers. Passenger locomotives had two axle leading bogies, fewer axles, and very large drivers in order to limit the speed at which the reciprocating parts had to move.
In the 1920s the focus in the United States turned to horsepower, epitomised by the "super power" concept promoted by the Lima Locomotive Works. Freight trains were to be driven faster; passenger trains needed to pull heavier loads at speed. In essence, the grate and firebox expanded without changes to the remainder of the locomotive, forcing the trailing bogie to grow a second axle. Freight 2-8-2s became 2-8-4s while 2-10-2s became 2-10-4s. Similarly, passenger 4-6-2s became 4-6-4s. In the United States this led to a convergence on the dual-purpose 4-8-4 configuration, which was used for both freight and passenger service.[13] Mallet locomotives went through a similar transformation and were upgraded from helpers into huge road engines with gargantuan fireboxes; their drivers increased in size in order to allow faster running.
The end of steam
The introduction of diesel-electric locomotives in the first part of the 20th century spelled the end of steam locomotives, though they were used in North America and Europe to mid-century, and continued in use in other countries to the end of the century. Steam locomotives are in general simple machines, which can be maintainable under primitive conditions and consume a wide variety of fuels. They are as a rule inefficient compared to modern diesels, requiring constant maintenance and labour to keep them operational.[14] Water is required at many points throughout a rail network and becomes a major problem in desert areas, as are found in some regions within the United States, Australia and South Africa. In other localities the local water is unsuitable. The reciprocating mechanism on the driving wheels tend to pound the rails (see "hammer blow"), thus requiring more maintenance. Steam locomotives require several hours' boiling up before service and an end-of-day procedure to remove ash and clinker. Diesel or electric locomotives, by comparison, commence working from the first turn of the key and do not require the labour-intensive cleaning, raking and servicing after a shift.[15] Finally, the smoke from steam locomotives is objectionable; in fact, the first electric and diesel locomotives were developed to meet smoke abatement requirements.[15]
United States
Mainline diesel-electric locomotives first appeared on the Baltimore and Ohio Railroad, in 1935 as locomotive No. 50. The diesel reduced maintenance costs dramatically, while increasing locomotive availability. On the Chicago, Rock Island and Pacific Railroad the new units delivered over 350,000 miles a year, compared with about 120,000–150,000 for a mainline steam locomotive.[5] World War II delayed dieselisation in the U.S.A, but the pace picked up in the 1950s, and by 1960 the last American Class I holdout, the Norfolk and Western Railway, discontinued steam operations. Some U.S. shortlines continued steam operations into the 1960s, and one steel mill continued to operate steam locomotives up to 1980.[Northwestern Steel and Wire][citation needed]
United Kingdom
Trials of diesel locomotives began in the United Kingdom in the 1930s but made only limited progress. One problem was that British diesel locomotives were often seriously under-powered, compared with the steam locomotives they were competing against.
After 1945, problems associated with post-war reconstruction and the availability of cheap domestic-produced coal kept steam in widespread use until the 1960s, when rising labour costs led to its withdrawal in 1968.[citation needed]. At the end of steam, British Railways estimated that its steam locomotives were costing around four times more in running costs than diesels (even though most of its steam locomotives were allowed to deteriorate to a sorry state of repair before being scrapped).[citation needed] The use of steam locomotives in British industry continued until 1974.[citation needed]
South Korea
In South Korea, the first steam locomotive was the Moga 2-6-0, followed by; Sata, Pureo, Ame, Sig, Mika, Pasi, Hyeogi, Class 901, Mateo, Sori, and Tou. Used until 1967, that train is now in the Railroad Museum.
Other countries
In other countries, the conversion from steam was slower. By March 1973 in Australia, steam had vanished in all states. Diesel locomotives were more efficient and the demand for manual labour to service and repairs was less than steam. Cheap oil had cost advantages over coal.
In the USSR, the last steam locomotive (model П36, serial number 251) was built in 1956; now in the Museum of Railway Machinery at former Warsaw Rail Terminal, Saint Petersburg, Russia. In the European part of the USSR, almost all steam locomotives were replaced by diesel and electrical ones in 1960s; in Siberia, steam locomotives were in active use till mid-1970s. However, many photographs exist of Russian steam locomotives at work into the late 1980's, some as late as 1989-1992, and it is known that until 1994, Russia had at least 1,000 steam locomotives stored in operable condition in case of "national emergencies". More than 200 steam locomotives are still in working conditions due to this precaution. However, it is possible that steam power returned to the eastern portion of Russia as late as 1998, due to a report from the "International Workings of Steam-2007" that a portion of the Trans-Siberian Express was steam hauled:
"In late 1998 I was told that 'Steam was being used again on the Trans-Siberian Express'. It sounded too good to be true so I ignored it. When I was in Bago (Myanmar) in late January 1999, I got talking to a Dutch couple who had recently been across Russia (and on through Mongolia to China) and they confirmed they had steam haulage for part of the way."
Strategic reserves of steam were confirmed as late as 2003 on the Krasnoyarsk Railway as well.
In Finland, the first diesels were introduced in the mid-1950s and they superseded the steam locomotives during the early '60s. The State Railways (VR) operated steam locomotives until 1975.
In South Africa an oil embargo combined with an abundance of cheap local coal, cheap labour force, ensured steam locomotives survived into the 1990s.[citation needed] Locomotive engineer L. D. Porta's designs appeared on a Class 19D engine in 1979, then a former Class 25 4-8-4 engine, became a Class 26, termed the "Red Devil" No. 3450, which demonstrated an improved overall performance with decreased coal and water consumption. The single class 26 locomotive operated until the end of steam. Another class 25NC locomotive, No. 3454, nicknamed the "Blue Devil" because of its colour scheme, received modifications including a most obvious set of double side-by-side exhaust stacks. In southern Natal, two former South African Railway Template:2ft gauge NGG16 Garratts operating on the privatised Port Shepstone & Alfred County Railway (ACR)received some L. D. Porta modifications in 1990 becoming a new NGG16A class.[16]
China continued to build mainline steam locomotives until late in the century, even building a few examples for American tourist operations. Since China was the last main-line user of steam locomotives, ending officially at the beginning of 2006, it is plausible that many still exist in industrial operations or in more remote parts of China. The last steam locomotives built in China were 2-10-2's in the late 1990's.[citation needed]
Hopes of revival
Dramatic increases in the cost of diesel fuel prompted several initiatives to revive steam power.[17][18] None of these has progressed to the point of production, and in the early 21st century, the steam locomotives operate only in a few isolated regions and in tourist operations.
In Germany a small number of fireless steam locomotives are still working in industrial service, e.g. at power stations.
The Swiss company Dampflokomotiv und Maschinenfabrik DLM AG delivered several new steam locomotives to rack railways in Switzerland and Austria between 1992 and 1996. One was the Brienz Rothorn Bahn.
References
- ^ a b Payton, Philip (2004): Oxford Dictionary of National Biography. Oxford University Press.
- ^ a b Ellis, Hamilton (1968). The Pictorial Encyclopedia of Railways. The Hamlyn Publishing Group. pp. pp.24-30.
{{cite book}}
:|pages=
has extra text (help) - ^ Swengel p. 146.
- ^ "National Museum of American History article on locomotive crews".
- ^ a b c d e f Bell, A Morton (1950): Locomotives, seventh edition. Virtue & Co Ltd, London.
- ^ a b c Snell, John B (1971): Mechanical Engineering: Railways. Longman, London.
- ^ a b Adams, Henry (1907): Cassell’s Engineers’ Handbook. Cassell and Co, London.
- ^ a b c d e Swengel, Frank M (1967). The American Steam Locomotive, Vol.1, The Evolution of the Steam Locomotive. Davenport, IA: MidWest Rail Publications.
{{cite book}}
: Cite has empty unknown parameter:|coauthors=
(help) - ^ U.S. National Parks Service online history resource: Pennsylvania Railroad chemical laboratory. Accessed 9 November 2006.
- ^ Unknown author (1957): Handbook for Railway Steam Locomotive Enginemen. British Transport Commission.
- ^ Oxford English Dictionary: Buff 1
- ^ Section 10, Railway Regulation Act, 1842. Her Majesty's Stationery Office.
- ^ Allen, Cecil J (1949): Locomotive Practice and Performance in the Twentieth Century. W Heffer and Sons Ltd, Cambridge, England.
- ^ "1935 article on the advantages of diesel locomotives".
- ^ a b Diesel Traction Manual for Enginemen, 15-16. British Transport Commission, 1962.
- ^ VidRail Productions, South African end of Steam: Orange Free State, Part 4, Vols. 3, 4 and 5 and Natal, Part 3, Vol. 1, in The Best of Southern African Steam, 1983-1990
- ^ "The 5AT project to develop a modern steam locomotive for British railways".
- ^ "Railway Extension Across the Andes: reactivation and modernisation of existing fleet of 75cm gauge 2-10-2 steam locomotives".
See also
- Steam engine
- Locomotive
- History of rail transport
- Steam locomotive nomenclature
- Geared steam locomotive
- High pressure steam locomotive
- Steam turbine locomotive
- Steam dummy
- List of heritage railways
- Live steam
- Steam locomotive components (contains detailed explanatory diagrams)
- Firetube boiler
- Steam locomotive production
- Stephenson's Rocket
- LMR 57 Lion Britain's oldest operable steam locomotive
- John Bull (locomotive) oldest operable steam locomotive in the world
- Dieselization
- Beyer-Garratt
Manufacturers
External links
- Database of surviving steam locomotives in North America
- Information on North American steam railroads in operation
- UK heritage railways and preserved locomotives database
- Pages for the British project to build a modern steam locomotive. The Advanced Steam Locomotive. (5AT)
- International Steam Locomotives
Books on steam locomotives
- C. E. Wolff, Modern Locomotive Practice: A Treatise on the Design, Construction, and Working of Steam Locomotives (Manchester, England, 1903)
- Henry Greenly, Model Locomotive (New York, 1905)
- G. R. Henderson, Cost of Locomotive Operation (New York, 1906)
- W. E. Dalby, Economical Working of Locomotives (London, 1906)
- A. I. Taylor, Modern British Locomotives (New York, 1907)
- E. L. Ahrons, The Development of British Locomotive Design (London, 1914)
- E. L. Ahrons, Steam Engine Construction and Maintenance (London, 1921)
- J. F. Gairns, Locomotive Compounding and Superheating (Philadelphia, 1907)
- Angus Sinclair, Development of the Locomotive Engine (New York, 1907)
- Vaughn Pendred, The Railway Locomotive, What it is and Why it is What it is (London, 1908)
- Brosius and Koch, Die Schule des Lokomotivführers (thirteenth edition, three volumes, Wiesbaden, 1909-1914)
- G. L. Fowler, Locomotive Breakdowns, Emergencies, and their Remedies (seventh edition, New York, 1911)
- Fisher and Williams, Pocket Edition of Locomotive Engineering (Chicago, 1911)
- T. A. Annis, Modern Locomotives (Adrian Michigan, 1912)
- C. E. Allen, Modern Locomotive (Cambridge, England, 1912)
- W. G. Knight, Practical Questions on Locomotive Operating (Boston, 1913)
- G. R. Henderson, Recent Development of the Locomotive (Philadelphia, 1913)
- Wright and Swift (editors) Locomotive Dictionary (third edition, Philadelphia, 1913)
- Roberts and Smith, Practical Locomotive Operating (Philadelphia, 1913)
- E. Prothero, Railways of the World (New York, 1914)
- M. M. Kirkman, The Locomotive (Chicago, 1914)
- C. L. Dickerson, The Locomotive and Things You Should Know About it (Clinton, Illinois, 1914)
- P. W. B. Semmens, A. J. Goldfinch, How Steam Locomotives Really Work (Oxford University Press, USA, 2004) ISBN 0-19-860782-2
- Gerald A Dee, A Lifetime of Railway Photography in Photographer Profile, Train Hobby Publications, Studfield, 1998. (Australian steam)
- Leon Oberg, Locomotives of Australia, Reed, Sydney, 1975.
- Swengel, F. M. The American Steam Locomotive; Vol. 1. The Evolution of the American Steam Locomotive, Midwest Rail Publication, Iowa, 1967.