Railway electrification
![]() | This article may require copy editing for grammar, style, cohesion, tone, or spelling. (April 2008) |


A Railway electrification system supplies electrical energy to railway locomotives and multiple units so that they can operate without having an on-board prime mover. There are several different electrification systems in use throughout the world. Railway electrification has many advantages but requires heavy capital expenditure for installation.
Characteristics of Electric Traction
The main advantage of electric traction is a higher power-to-weight ratio than methods such as diesel or steam that generate power on board. This enables faster acceleration and higher tractive effort on steep grades. On locomotives equipped with regenerative braking, descending steep grades requires little use of air brakes as the locomotive's traction motors become generators sending current back into the supply system and/or on-board resistors (which convert the excess energy to heat).
Other advantages include the lack of exhaust fumes at point of use, less noise and lower maintenance requirements of the traction units. Given sufficient traffic density electric trains produce fewer carbon emissions than diesel trains, especially in countries where electricity comes primarily from non-fossil sources, such as Austria and France.
A fully electrified railway would have no need to switch between methods of traction thereby making operations more efficient. One country that approaches this ideal is Switzerland.
The main disadvantage is the capital cost of the electrification equipment, most significantly for long distance lines that do not generate heavy traffic. Suburban railways with closely-spaced stations and high traffic density are the most likely to be electrified, and main lines carrying heavy and frequent traffic are also electrified in many countries.
Classification

Electrification systems are classified by three main parameters:
- Current
- Direct current (DC)
- Alternating current (AC)
- Contact System
Direct current
Early electric systems used low-voltage DC. Electric motors were fed directly from the traction supply, and were controlled using a combination of resistors and relays that connected the motors in parallel or series.
The most common DC voltages are 600 V and 750 V for trams and metros, and 1500 V and 3000 V for railways. The lower voltages are often used with third or fourth rail systems, and voltages above 1000 V are normally limited to overhead wiring for safety reasons. The "S-Bahn" in Hamburg, Germany, has third rail with 1200 V, the French SNCF Culoz-Modane main line electrification in the Alps used 1500 V and a third rail until 1976, when a catenary was installed, and the third rail was disposed of.
During the middle 20th century, rotary converters or mercury arc rectifiers were used to convert utility (mains) AC power to the required DC voltage at the feeder stations. Today, this is usually done by semiconductor rectifiers after transforming the voltage down from the utility supply.
The DC system is quite simple, but it requires thick cables and short distances between feeder stations because of the heavy currents required. There are also significant resistive losses. In the UK, the maximum current that can be drawn by a train is 6800 A at 750 V[1]. The feeder stations require constant monitoring, and on many systems, only one train or locomotive is allowed per section. The distance between two feeder stations at 3000 V is about 25 km (15 miles).
If auxiliary machinery, such as fans and compressors, is powered by motors fed directly from the traction supply they may be larger because of the extra insulation required for the relatively high operating voltage. Alternatively, they can be powered from a motor-generator set, which was provided as an alternative way of powering incandescent lights which otherwise had to be connected as series strings (bulbs designed to operate at traction voltages being particularly inefficient). Now solid-state converters (SIVs) and fluorescent lights can be used.
Voltage
The permissible range of voltages allowed are as stated in standards BS EN 50163 and IEC 60850. These take into account the number of trains drawing current and their distance from the substation.
Electrification system | Lowest non-permanent voltage | Lowest permanent voltage | Nominal voltage | Highest permanent voltage | Highest non-permanent voltage |
---|---|---|---|---|---|
600 V DC | 400 V | 400 V | 600 V | 720 V | 800 V |
750 V DC | 500 V | 500 V | 750 V | 900 V | 1000 V |
1500 V DC | 1000 V | 1000 V | 1500 V | 1800 V | 1950 V |
3000 V DC | 2000 V | 2000 V | 3000 V | 3600 V | 3900 V |
15,000 V AC, 16⅔ Hz | 11,000 V | 12,000 V | 15,000 V | 17,250 V | 18,000 V |
25,000 V AC, 50 Hz | 17,500 V | 19,000 V | 25,000 V | 27,500 V | 29,000 V |
Overhead systems

1500 V DC is used in The Netherlands, Japan, Ireland, parts of Australia and France, and in Wellington, New Zealand. In the United States, 1500 V DC is used in the Chicago area on the Metra Electric district and the South Shore Line interurban line. In Slovakia, there are two narrow gauge lines in the High-Tatras (one a cog railway). In Portugal, it is used in the Cascais line, and in Denmark on the suburban S-train system.

In the United Kingdom, 1500 V DC was used in 1954 for the Woodhead trans-Pennine route (now closed); the system used regenerative braking, allowing for transfer of energy between climbing and descending trains on the steep approaches to the tunnel. The system was also used for suburban electrification in East London and Manchester, now converted to 25 kV AC.
3000 V DC is used in Belgium, Italy, Spain, Poland, the northern Czech Republic, Slovakia, Slovenia, western Croatia, South Africa and in the former Soviet Union. It was also formerly used by the Milwaukee Road's extensive electrification across the Continental Divide, and by the Delaware, Lackawanna & Western Railroad (now NJ Transit, converted to 25 kV AC).
Third rail



Most electrification systems use overhead wires, but third rail is an option up to about 1200 V. While use of a third rail does not require the use of DC, in practice all third-rail systems use DC because it can carry 41% more power than an AC system operating at the same peak voltage. Third rail is more compact than overhead wires and can be used in smaller-diameter tunnels, an important factor for subway systems.
Third rail systems can be designed to use top contact, side contact, or bottom contact. Top contact is less safe, as the live rail is exposed to people treading on the rail unless an insulating hood is provided. Side- and bottom-contact third rail can easily have safety shields incorporated, carried by the rail itself. Uncovered top-contact third rails are vulnerable to disruption caused by ice, snow, and fallen leaves.
DC systems are limited to relatively low voltages, and this can limit the size and speed of trains and the amount of air-conditioning the trains can provide. This may be a factor favouring overhead wires and high voltage AC, even for urban usage. In practice the top speed of trains on third-rail systems is limited to 100 mph (160 km/h) because above that speed reliable contact between the shoe and the rail cannot be maintained.
Some road operating trams (streetcars) used conduit third-rail current collection. The third rail was below street level. The tram picked up the current via a plough accessed through a narrow slot in the road. In the United States, the former trolley system in Washington, D.C. was operated in this manner to avoid the unsightly wires and poles associated with electric traction. The evidence of this mode of running can still be seen on the track down the slope on the northern access to the abandoned Kingsway Tramway Subway (in central London). The slot between the running rails is clearly visible. The slot can easily be confused with the similar looking slot for cable trams (indeed, in some cases, the conduit slot was originally a cable slot).
Fourth rail
The London Underground is one of the few networks that uses a four-rail system. The additional rail carries the electrical return that on third rail and overhead networks is provided by the running rails. On the London Underground a top-contact third rail is beside the track, energised at +420 V DC, and a top-contact fourth rail is located centrally between the running rails at -210 V DC, which combine to provide a traction voltage of 630 V DC. The same system was used for Milan's oldest underground line (Milan Metro line 1); the more recent lines use an overhead catenary.
This scheme was introduced because of the problems of return currents, intended to be carried by the earthed running rails, running through the iron tunnel linings instead. This can cause electrolytic damage and potential arcing if the segments aren't properly joined. The problem was exacerbated because the return current also had a tendency to flow through nearby iron water and gas mains. Some of these, particularly Victorian mains that predated London's underground railways, were never constructed to carry such currents. The four-rail system solves the problem. Although the supply is not isolated from earth for safety reasons, it is connected by resistors that ensure that stray earth currents are kept to manageable levels.
London's sub-surface underground railways also operate on the four-rail scheme, partly for compatibility with the electrical distribution system, but mainly for rolling stock movements.

On lines shared with National Rail third-rail stock, the centre 'negative' rail is connected to the return running rail, allowing both types of train to operate.
A system proposed (but not used) by the South Eastern and Chatham Railway around 1920 was 1,500 V DC four-rail. Technical details are scarce, but it is likely that it would have been a "mid-earth" system with one conductor rail at +750 volts and the other at -750 volts. This would have facilitated conversion to 750 V DC three-rail at a later date.
A few lines of the Paris Metro also operate on a four-rail power scheme, but for a very different reason. It is not strictly a four-rail scheme as they run on rubber tyres running on a pair of narrow roadways made of steel, and in some places, concrete. Since the tyres do not conduct the return current, two conductor rails are provided outside of the running 'roadways', so at least electrically it fits as a four-rail scheme. The trains are designed to operate from either polarity of supply, because some lines use reversing loops at one end, causing the train to be reversed during every complete journey (intended to save having to run the locomotive round).
Alternating current
These are overhead electrification systems.
Low-frequency alternating current
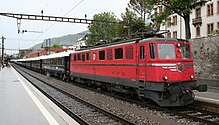
Common commutating electric motors can also be fed AC (universal motor), because reversing current in both stator and rotor does not change the direction of torque. However, inductance of the windings makes large motors impractical at standard AC distribution frequencies. Five European countries, including Germany, Austria, Switzerland, Norway, and Sweden have standardised on 15 kV 16⅔ Hz (one-third the normal mains frequency) single-phase AC (Germany, Austria and Switzerland used 6 kV and 7.5 kV systems until 1995). In the United States (with its 60 Hz distribution system), 25 Hz (an older, now-obsolete standard mains frequency) is used at 11 kV between Washington, DC and New York City. A 12.5 kV 25 Hz section between New York City and New Haven, Connecticut was converted to 60 Hz in the last third of the 20th century.
In the UK, the London, Brighton and South Coast Railway pioneered overhead electrification of its suburban lines in London. London Bridge to Victoria being opened to traffic on December 1 1909. Victoria to Crystal Palace via Balham and West Norwood opened in May 1911. Peckham Rye to West Norwood opened in June 1912. Further extensions were not made owing to the First World War. Two lines opened in 1925 under the Southern Railway serving Coulsdon North and Sutton railway station. It was announced in 1926 that all lines were to be converted to third rail electric and the last overhead electric service ran in September 1929. The lines were electrified at 6.7 kV, 25 Hz.[2][3][4]
In such a system, the traction motors can be fed through a transformer with multiple taps. Changing the taps allows the motor voltage to be changed without requiring power-wasting resistors. Auxiliary machinery is driven by low voltage commutating motors, powered from a separate winding of the main transformer, and are reasonably small.
The unusual frequency requires that electricity be converted from utility power by motor-generators or static inverters at the feeding substations, or generated at altogether separate traction powerstations.
Since 1979 the three phase induction motor has become almost universally used. It is fed by a static four quadrant converter which supplies a constant voltage current to a pulse width modulator inverter that supplies the three-phase variable frequency to the motors. This system has made the low frequency systems advantageous again, because of its inherent recuperation capability, something precluded by the phase-breaks in the industrial frequency systems.
Polyphase alternating current systems

The Italian State railway system was 3300 V at 15-16.7 Hz. With such a low frequency the locomotives did not need gearing. It is also possible to use the polyphase system regeneratively, as on the Italian State railway's mountain lines, where a loaded train descending could supply much of the power for a train ascending.
In the USA the Great Northern Railway's (Cascade Tunnel) first electrified line (1909-1927) was at 6600 V, 25 Hz.
The main complexity with three-phase systems is the need for two conductors. Italian State Railways used a wide bow collector which covered both wires. In the U.S., a pair of trolley poles were used. They worked well with a maximum speed limit of 15 mph. A dual conductor pantograph system is used on four mountain railways that continue to use three phase power (Corcovado Rack Railway in Rio de Janeiro, Brazil, Jungfraubahn and Gornergratbahn in Switzerland, and the Petit train de la Rhune in France).
Standard frequency alternating current
Only in the 1950s after development in France did the standard frequency single-phase alternating current system become widespread, despite the simplification of a distribution system which could use the existing power supply network.
The first attempts to use standard-frequency single-phase AC were made in Hungary in the 1930s, by the Hungarian Kálmán Kandó on the line between Budapest-Nyugati and Alag, using 16 kV at 50 Hz. The locomotives carried a four-pole rotating phase converter feeding a single traction motor of the polyphase induction type at 600 to 1100 volts. The number of poles on the 2,500 HP motor could be changed using slip rings to run at one of four synchronous speeds.
Today, some locomotives in this system use a transformer and rectifier that provide low-voltage pulsating DC current to motors. Speed is controlled by switching winding taps on the transformer. More sophisticated locomotives use thyristor or IGBT transistor circuitry to generate chopped or even variable-frequency AC that is then directly consumed by AC traction motors.
This system is quite economical, but it has its drawbacks: the phases of the external power system are loaded unequally, and there is significant electromagnetic interference generated, not to mention acoustic noise.
A list of the countries using the 25 kV, 50 Hz single-phase AC system can be found in the list of current systems for electric rail traction.

The United States commonly uses 12.5 and 25 kV at 25 Hz or 60 Hz. 25 kV AC is the preferred system for new high speed and long distance railways, even if the railway uses a different system for existing trains.
To prevent the risk of out of phase supplies mixing, sections of line fed from different feeder station must be kept strictly isolated. This is achieved by Neutral Sections (also known as Phase Breaks), usually provided at feeder stations and midway between them, although typically only half are in use at any time, the others being provided to allow a feeder station to be shut down and power provided from adjacent feeder stations. Neutral Sections usually consist of an earthed section of wire which is separated from the live wires on either side by insulating material, typically ceramic beads, designed so the pantograph will smoothly run from one section to the other. The earthed section prevents an arc being drawn from one live section to the other, as the voltage difference may be higher than the normal system voltage if the live sections are on different phases, and the protective circuit breakers may not be able to safety interrupt the considerable current that would flow. To prevent the risk of an arc being drawn across from one section of wire to earth, when passing through the neutral section the train must be coasting and the circuit breakers must be open. In many cases, this is done manually by the driver. To help them, a warning board is provided just before both the neutral section and an advanced warning some distance before. A further board is then provided after the neutral section to tell the driver they can reclose the circuit breaker, although the driver must not do this until the rear pantograph has passed this board. In the UK, a system known as Automatic Power Control (APC) automatically opens and closes the circuit breaker, this being achieved by using sets of permanent magnets alongside the track communicating with a detector on the train. The only action needed by the driver is to shut off power and coast, and therefore warning boards are still provided at and on the approach to neutral sections.
On French LGV lines, the UK High Speed 1 Channel Tunnel rail link and in the Channel Tunnel itself, neutral sections are negotiated automatically.
References
- ^ Technical specification for interoperability relating to the energy subsystem of the trans-European high-speed rail system
- ^ Southern Electric
- ^ History of Southern Electrification Part 1
- ^ History of Southern Electrification Part 2
Sources
- Moody, G T (1960). "Part One". Southern Electric (3rd edition ed.). London: Ian Allan Ltd.
{{cite book}}
:|edition=
has extra text (help); Cite has empty unknown parameters:|coauthors=
and|month=
(help)
See also
|