Thorium
![]() | |||||||||||||||||||||||||||||||||||||||||||||||||||
Thorium | |||||||||||||||||||||||||||||||||||||||||||||||||||
---|---|---|---|---|---|---|---|---|---|---|---|---|---|---|---|---|---|---|---|---|---|---|---|---|---|---|---|---|---|---|---|---|---|---|---|---|---|---|---|---|---|---|---|---|---|---|---|---|---|---|---|
Pronunciation | /ˈθɔːriəm/ | ||||||||||||||||||||||||||||||||||||||||||||||||||
Appearance | silvery | ||||||||||||||||||||||||||||||||||||||||||||||||||
Standard atomic weight Ar°(Th) | |||||||||||||||||||||||||||||||||||||||||||||||||||
Thorium in the periodic table | |||||||||||||||||||||||||||||||||||||||||||||||||||
| |||||||||||||||||||||||||||||||||||||||||||||||||||
Atomic number (Z) | 90 | ||||||||||||||||||||||||||||||||||||||||||||||||||
Group | f-block groups (no number) | ||||||||||||||||||||||||||||||||||||||||||||||||||
Period | period 7 | ||||||||||||||||||||||||||||||||||||||||||||||||||
Block | f-block | ||||||||||||||||||||||||||||||||||||||||||||||||||
Electron configuration | [Rn] 6d2 7s2 | ||||||||||||||||||||||||||||||||||||||||||||||||||
Electrons per shell | 2, 8, 18, 32, 18, 10, 2 | ||||||||||||||||||||||||||||||||||||||||||||||||||
Physical properties | |||||||||||||||||||||||||||||||||||||||||||||||||||
Phase at STP | solid | ||||||||||||||||||||||||||||||||||||||||||||||||||
Melting point | 2023 K (1750 °C, 3182 °F) | ||||||||||||||||||||||||||||||||||||||||||||||||||
Boiling point | 5061 K (4788 °C, 8650 °F) | ||||||||||||||||||||||||||||||||||||||||||||||||||
Density (at 20° C) | 11.725 g/cm3 [3] | ||||||||||||||||||||||||||||||||||||||||||||||||||
Heat of fusion | 13.81 kJ/mol | ||||||||||||||||||||||||||||||||||||||||||||||||||
Heat of vaporization | 514 kJ/mol | ||||||||||||||||||||||||||||||||||||||||||||||||||
Molar heat capacity | 26.230 J/(mol·K) | ||||||||||||||||||||||||||||||||||||||||||||||||||
Vapor pressure
| |||||||||||||||||||||||||||||||||||||||||||||||||||
Atomic properties | |||||||||||||||||||||||||||||||||||||||||||||||||||
Oxidation states | common: +4 −1,[4] +1,? +2,[5] +3[5] | ||||||||||||||||||||||||||||||||||||||||||||||||||
Electronegativity | Pauling scale: 1.3 | ||||||||||||||||||||||||||||||||||||||||||||||||||
Ionization energies |
| ||||||||||||||||||||||||||||||||||||||||||||||||||
Atomic radius | empirical: 179.8 pm | ||||||||||||||||||||||||||||||||||||||||||||||||||
Covalent radius | 206±6 pm | ||||||||||||||||||||||||||||||||||||||||||||||||||
![]() | |||||||||||||||||||||||||||||||||||||||||||||||||||
Other properties | |||||||||||||||||||||||||||||||||||||||||||||||||||
Natural occurrence | primordial | ||||||||||||||||||||||||||||||||||||||||||||||||||
Crystal structure | face-centered cubic (fcc) (cF4) | ||||||||||||||||||||||||||||||||||||||||||||||||||
Lattice constant | a = 508.45 pm (at 20 °C)[3] | ||||||||||||||||||||||||||||||||||||||||||||||||||
Thermal expansion | 11.54×10−6/K (at 20 °C)[3] | ||||||||||||||||||||||||||||||||||||||||||||||||||
Thermal conductivity | 54.0 W/(m⋅K) | ||||||||||||||||||||||||||||||||||||||||||||||||||
Electrical resistivity | 157 nΩ⋅m (at 0 °C) | ||||||||||||||||||||||||||||||||||||||||||||||||||
Magnetic ordering | paramagnetic[6] | ||||||||||||||||||||||||||||||||||||||||||||||||||
Molar magnetic susceptibility | 132.0×10−6 cm3/mol (293 K)[7] | ||||||||||||||||||||||||||||||||||||||||||||||||||
Young's modulus | 79 GPa | ||||||||||||||||||||||||||||||||||||||||||||||||||
Shear modulus | 31 GPa | ||||||||||||||||||||||||||||||||||||||||||||||||||
Bulk modulus | 54 GPa | ||||||||||||||||||||||||||||||||||||||||||||||||||
Speed of sound thin rod | 2490 m/s (at 20 °C) | ||||||||||||||||||||||||||||||||||||||||||||||||||
Poisson ratio | 0.27 | ||||||||||||||||||||||||||||||||||||||||||||||||||
Mohs hardness | 3.0 | ||||||||||||||||||||||||||||||||||||||||||||||||||
Vickers hardness | 295–685 MPa | ||||||||||||||||||||||||||||||||||||||||||||||||||
Brinell hardness | 390–1500 MPa | ||||||||||||||||||||||||||||||||||||||||||||||||||
CAS Number | 7440-29-1 | ||||||||||||||||||||||||||||||||||||||||||||||||||
History | |||||||||||||||||||||||||||||||||||||||||||||||||||
Naming | after Thor, the Norse god of thunder | ||||||||||||||||||||||||||||||||||||||||||||||||||
Discovery | Jöns Jakob Berzelius (1829) | ||||||||||||||||||||||||||||||||||||||||||||||||||
Isotopes of thorium | |||||||||||||||||||||||||||||||||||||||||||||||||||
| |||||||||||||||||||||||||||||||||||||||||||||||||||
Thorium is a naturally occurring radioactive chemical element with the symbol Th and atomic number 90. It was discovered in 1828 by the Norwegian mineralogist Morten Thrane Esmark and identified by the Swedish chemist Jöns Jakob Berzelius and named after Thor, the Norse god of thunder.
Thorium produces a radioactive gas, radon-220, as one of its decay products. Secondary decay products of thorium include radium and actinium. In nature, virtually all thorium is found as thorium-232, which undergoes alpha decay with a half-life of about 14.05 billion years. Other isotopes of thorium are short-lived intermediates in the decay chains of higher elements, and only found in trace amounts. Thorium is estimated to be about three to four times more abundant than uranium in the Earth's crust, and is chiefly refined from monazite sands as a by-product of extracting rare earth metals.
Thorium was once commonly used as the light source in gas mantles and as an alloying material, but these applications have declined due to concerns about its radioactivity. Thorium is also used as an alloying element in nonconsumable TIG welding electrodes. It remains popular as a material in high-end optics and scientific instrumentation; thorium and uranium are the only radioactive elements with major commercial applications that do not rely ass on their radioactivity.
Canada, China, Germany, India, the Netherlands, the United Kingdom and the United States have experimented with using thorium as a substitute nuclear fuel in nuclear reactors.[10] When compared to uranium, there is a growing interest in thorium-based nuclear power due to its greater safety benefits, absence of non-fertile isotopes and its higher occurrence and availability.[10] India's three stage nuclear power programme is possibly the most well known and well funded of such efforts.[11][12]
Characteristics
Physical properties
Pure thorium is a silvery-white metal that is air-stable and retains its luster for several months. When contaminated with the oxide, thorium slowly tarnishes in air, becoming gray and finally black. The degree of oxide contamination greatly influences thorium's physical properties.[13]
The purest specimens often contain several tenths of a percent of the oxide. Pure thorium is soft, very ductile, and can be cold-rolled, swaged, and drawn. Thorium is dimorphic, changing at 1360 °C from a face-centered cubic to a body-centered cubic structure; a body-centered tetragonal lattice form exists at high pressure with impurities driving the exact transition temperatures and pressures.[13]
Powdered thorium metal is often pyrophoric and requires careful handling. When heated in air, thorium metal turnings ignite and burn brilliantly with a white light. Thorium has one of the largest liquid temperature ranges of any element, with 2946 °C between the melting point and boiling point.[14] Thorium metal is paramagnetic with a ground state of 6d27s2.[13]
Chemical properties
Thorium is slowly attacked by water, but does not dissolve readily in most common acids, except hydrochloric acid.[14] It dissolves in concentrated nitric acid containing a small amount of catalytic fluoride ion.[15]
Thorium's oxide is ThO2. Thorium's most common oxidation state is +4, as in ThF4, but thorium also has an oxidation state of +3, as in ThI3. Thorium has been shown to activate carbon-hydrogen bonds, forming unusual compounds. Thorium atoms can also bond to more atoms than any other element. For instance, in the compound thorium tetrakisaminodiborane thorium bonds to fifteen hydrogen atoms.[16]
Compounds
Thorium compounds are stable in the +4 oxidation state.[17]
Thorium dioxide has the highest melting point (3300 °C) of all oxides.[18]
Thorium(IV) nitrate and thorium(IV) fluoride are known in their hydrated forms: Th(NO
3)
4·4H
2O and ThF
4·4H
2O, respectively.[17] Thorium(IV) carbonate, Th(CO
3)
2, is also known.[17]
When treated with potassium fluoride and hydrofluoric acid, Th4+ forms the complex anion ThF2−
6, which precipitates as an insoluble salt, K
2ThF
6.[15]
Thorium(IV) hydroxide, Th(OH)
4, is highly insoluble in water, and is not amphoteric. The peroxide of thorium, ThO4 or Th(O2)2, is rare in being an insoluble solid. This property can be used to separate thorium from other ions in solution.[15]
In the presence of phosphate anions, Th4+ forms precipitates of various compositions, which are insoluble in water and acid solutions.[15]
Thorium monoxide has recently been produced through laser ablation of thorium in the presence of oxygen.[19] This highly polar molecule has the largest known internal electric field.[20]
Isotopes
Twenty-seven radioisotopes have been characterized, with a range in atomic weight from 210 u (210Th) to 236 u (236Th).[21] The most stable isotopes are:
- 232Th with a half-life of 14.05 billion years, it represents all but a trace of naturally occurring thorium.
- 230Th with a half-life of 75,380 years. Occurs as the daughter product of 238U decay.
- 229Th with a half-life of 7340 years. It has a nuclear isomer (or metastable state) with a remarkably low excitation energy of 7.6 eV.[22]
- 228Th with a half-life of 1.92 years.
All of the remaining radioactive isotopes have half-lives that are less than thirty days and the majority of these have half-lives that are less than ten minutes.
Applications
Thorium is a component of the magnesium alloy series, called Mag-Thor, used in aircraft engines and rockets and imparting high strength and creep resistance at elevated temperatures.[23][24] Thoriated magnesium was used to build the CIM-10 Bomarc missile, although concerns about radioactivity have resulted in several missiles being removed from public display.
Thorium is also used in its oxide form (thoria) in gas tungsten arc welding (GTAW) to increase the high-temperature strength of tungsten electrodes and improve arc stability.[25] The electrodes labeled EWTH-1 contain 1% thoria, while the EWTH-2 contain 2%.[26] In electronic equipment, thorium coating of tungsten wire improves the electron emission of heated cathodes.[14]
Thorium is a very effective radiation shield, although it has not been used for this purpose as much as lead or depleted uranium. Uranium-thorium age dating has been used to date hominid fossils,[14] seabeds, and mountain ranges.[27]
Environmental concerns related to radioactivity led to a sharp decrease in demand for nonnuclear uses of thorium in the 2000s.[27]
Thorium compounds

Thorium dioxide (ThO2) and thorium nitrate (Th(NO
3)
4) were used in mantles of portable gas lights, including natural gas lamps, oil lamps and camping lights. These mantles glow with an intense white light (unrelated to radioactivity) when heated in a gas flame, and its color could be shifted to yellow by addition of cerium.[24]
Thorium dioxide is a material for heat-resistant ceramics, e.g., for high-temperature laboratory crucibles.[25] When added to glass, it helps increase refractive index and decrease dispersion. Such glass finds application in high-quality lenses for cameras and scientific instruments.[14] The radiation from these lenses can self-darken (yellow) them over a period of years and degrade film, but the health risks are minimal.[28] Yellowed lenses may be restored to their original colorless state with lengthy exposure to intense UV light.
Thorium dioxide was used to control the grain size of tungsten metal used for spirals of electric lamps. Thoriated tungsten elements are found in the filaments of magnetron tubes. Thorium is added because of its ability to emit electrons at relatively low temperatures when heated in vacuum. Those tubes generate microwave frequencies and are applied in microwave ovens and radars.[24]
Thorium dioxide has been used as a catalyst in the conversion of ammonia to nitric acid,[25] in petroleum cracking and in producing sulfuric acid. It is the active ingredient of Thorotrast, which was used as radiocontrast agent for X-ray diagnostics. This use has been abandoned due to its carcinogenic nature.[14]
Despite its radioactivity, thorium fluoride (ThF4) is used as an antireflection material in multilayered optical coatings. It has excellent optical transparency in the range 0.35–12 µm, and its radiation is primarily due to alpha particles, which can be easily stopped by a thin cover layer of another material.[29] Thorium fluoride was also used in manufacturing carbon arc lamps, which provided high-intensity illumination for movie projectors and search lights.[24]
Thorium as a nuclear fuel
Benefits and challenges
The naturally occurring isotope thorium-232 is a fertile material, and with a suitable neutron source can be used as nuclear fuel in nuclear reactors, including breeder reactors. In 1997, the U.S. Energy Department underwrote research into thorium fuel, and research also was begun in 1996 by the International Atomic Energy Agency (IAEA), to study the use of thorium reactors. Nuclear scientist Alvin Radkowsky of Tel Aviv University in Israel founded a consortium to develop thorium reactors, which included other companies: Raytheon Nuclear Inc., Brookhaven National Laboratory and the Kurchatov Institute in Moscow.[30]
Radkowsky was chief scientist in the U.S. nuclear submarine program directed by Admiral Hyman Rickover and later headed the design team that built the USA's first civilian nuclear power plant at Shippingport, Pennsylvania, which was a scaled-up version of the first naval reactor.[30] The third Shippingport core, initiated in 1977, bred thorium.[31] Even earlier examples of reactors using fuel with thorium exist, including the first core at the Indian Point Energy Center in 1962.[32]
Some countries, including India, are now investing in research to build thorium-based nuclear reactors. A 2005 report by the International Atomic Energy Agency discusses potential benefits along with the challenges of thorium reactors.[33] India has also made thorium-based nuclear reactors a priority with its focus on developing fast breeder technology.[34][35]
Some benefits of thorium fuel when compared with uranium were summarized as follows:[36]
- Weapons-grade fissionable material (233U) is harder to retrieve safely and clandestinely from a thorium reactor;
- Thorium mining produces a single pure isotope, whereas the mixture of natural uranium isotopes must be enriched to function in most common reactor designs. The same cycle could also use the fissionable U-238 component of the natural uranium, and also contained in the depleted reactor fuel;
- Thorium cannot sustain a nuclear chain reaction without priming,[37] so fission stops by default in an accelerator driven reactor.
When used in a breeder-like reactor, however, unlike uranium-based light water reactors, thorium requires irradiation and reprocessing before the above-noted advantages of thorium-232 can be realized, which initially makes solid thorium fuels more expensive than uranium fuels.[27] But experts note that "the second thorium reactor may activate a third thorium reactor. This could continue in a chain of reactors for a millennium if we so choose." They add that because of thorium's abundance, it will not be exhausted in 1,000 years.[38]
The Thorium Energy Alliance (TEA), an educational advocacy organization, emphasizes that "there is enough thorium in the United States alone to power the country at its current energy level for over 10,000 years."[39]
Thorium energy fuel cycle
"Thorium is like wet wood […it] needs to be turned into fissile uranium just as wet wood needs to be dried in a furnace."
Like 238U, 232Th is not fissile itself, but it is fertile: it absorbs slow neutrons to produce, after two beta decays, 233U, which is fissile.[27] The preparation of thorium fuel does not require isotopic separation, unlike the preparation of uranium fuels.
The thorium fuel cycle creates 233U, which, if separated from the reactor's fuel, could with some difficulty be used for making nuclear weapons. This is one reason why a liquid-fuel cycle (e.g., molten salt reactor or MSR) is preferred—only a limited amount of 233U ever exists in the reactor and its heat-transfer systems, preventing access to weapons material. However, the neutrons produced by the reactor can be absorbed by a thorium or uranium blanket to produce fissile 233U or 239Pu. Also, the 233U could be continuously extracted from the molten fuel as the reactor runs. Neutrons from the decay of uranium-233 can be fed back into the fuel cycle to start the cycle again.[27]
The neutron flux from spontaneous fission of 233U is negligible. 233U can thus be used easily in a simple gun-type nuclear bomb design.[41] In 1977, a light-water reactor at the Shippingport Atomic Power Station was used to establish a 232Th-233U fuel cycle. The reactor worked until its decommissioning in 1982.[42][43][44] Thorium can be and has been used to power nuclear energy plants using both the modified traditional Generation III reactor design and prototype Generation IV reactor designs. The use of thorium as an alternative fuel is one innovation being explored by the International Project on Innovative Nuclear Reactors and Fuel Cycles (INPRO),[45] conducted by the International Atomic Energy Agency (IAEA).
Unlike its use in Molten salt reactors, when using solid thorium in modified light water reactor (LWR) problems include: the undeveloped technology for fuel fabrication; in traditional, once-through LWR designs potential problems in recycling thorium due to highly radioactive 228Th; some weapons proliferation risk due to production of 233U; and the technical problems (not yet satisfactorily solved) in reprocessing. Much development work is still required before the thorium fuel cycle can be commercialized for use in LWR. The effort required has not seemed worth it while abundant uranium is available.
Commercial nuclear power station
India's Kakrapar-1 reactor is the world's first reactor that uses thorium rather than depleted uranium for power flattening across the reactor core.[46] India, which has about 25% of the world's thorium reserves, is developing a 300 MW prototype of a thorium-based Advanced Heavy Water Reactor (AHWR). The prototype is expected to be fully operational by 2016,[47] after which they plan to construct five more reactors.[48][49] The reactor is a fast breeder reactor and uses a plutonium core rather than an accelerator to produce neutrons. As accelerator-based systems can operate at sub-criticality they could be developed too, but that would require more research.[50] India currently envisages meeting 30% of its electricity demand through thorium-based reactors by 2050.[51]
Existing thorium energy projects
While research is under way in many countries, only India is building utility-scale plants, mostly planned to thorium-fueled. In 2012, the first commercial fast reactor capable of using thorium was allegedly nearing completion, according to Srikumar Banerjee, former Chairman of the Indian Atomic Energy Commission.[52]
Projects combining uranium and thorium
Fort St. Vrain Generating Station, a demo HTGR in Colorado, USA, operating from 1977 until 1992, employed enriched uranium fuel that also contained thorium. This resulted in high fuel efficiency because the thorium was converted to uranium and then fissioned.
History

Morten Thrane Esmark found a black mineral on Løvøya island, Norway, and gave a sample to his father, Jens Esmark, a noted mineralogist. The elder Esmark was not able to identify it and sent a sample to the Swedish chemist Jöns Jakob Berzelius for examination in 1828. Berzelius determined that it contained a new element, which he named thorium after Thor, the Norse god of thunder.[25] He published his findings in 1829.[53][54][55] Berzelius reused the name of a previous element discovery from a mineral from the Falun, which later proved to be a yttrium mineral.[53][56] The metal had no practical uses until Carl Auer von Welsbach invented the gas mantle in 1885.[25]
Thorium was first observed to be radioactive in 1898, independently, by the Polish-French physicist Marie Curie and the German chemist Gerhard Carl Schmidt.[57][58][59] Between 1900 and 1903, Ernest Rutherford and Frederick Soddy showed how thorium decayed at a fixed rate over time into a series of other elements. This observation led to the identification of half-life as one of the outcomes of the alpha particle experiments that led to their disintegration theory of radioactivity.[60]
The crystal bar process (or "iodide process") was discovered by Anton Eduard van Arkel and Jan Hendrik de Boer in 1925 to produce high-purity metallic thorium.[61]
The name ionium was given early in the study of radioactive elements to the 230Th isotope produced in the decay chain of 238U before it was realized that ionium and thorium were chemically identical. The symbol Io was used for this supposed element.

Thorium-232 is a primordial nuclide, having existed in its current form for over 4.5 billion years, predating the formation of the Earth; it was forged in the cores of dying stars through the r-process and scattered across the galaxy by supernovas.[62] Its radioactive decay produces a significant amount of the Earth's internal heat.[63]
Occurrence

Thorium is found in small amounts in most rocks and soils; it is three times more abundant than tin in the Earth's crust and is about as common as lead.[64] Soil commonly contains an average of around 6 parts per million (ppm) of thorium.[65] Thorium occurs in several minerals including thorite (ThSiO4), thorianite (ThO2 + UO2) and monazite. Thorianite is a rare mineral and may contain up to about 12% thorium oxide. Monazite contains 2.5% thorium, allanite has 0.1 to 2% thorium and zircon can have up to 0.4% thorium.[66] Thorium-containing minerals occur on all continents.[14][67][68] Thorium is several times more abundant in Earth's crust than all isotopes of uranium combined and thorium-232 is several hundred times more abundant than uranium-235.[27]
Thorium concentrations near the surface of the earth can be mapped using gamma spectroscopy. The same technique has been used to detect concentrations on the surface of the moon; the near side has high abundances of relatively Thorium-rich KREEP, while the Compton–Belkovich Thorium Anomaly was detected on the far side. Martian thorium has also been mapped by 2001 Mars Odyssey.[69]
232Th decays very slowly (its half-life is comparable to the age of the universe) but other thorium isotopes occur in the thorium and uranium decay chains. Most of these are short-lived and hence much more radioactive than 232Th, though on a mass basis they are negligible.
Extraction
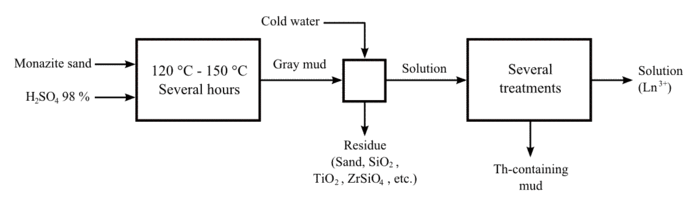

Thorium has been extracted chiefly from monazite through a complex multi-stage process. The monazite sand is dissolved in hot concentrated sulfuric acid (H2SO4). Thorium is extracted as an insoluble residue into an organic phase containing an amine. Next it is separated or stripped using an ion such as nitrate, chloride, hydroxide, or carbonate, returning the thorium to an aqueous phase. Finally, the thorium is precipitated and collected.[70]
Several methods are available for producing thorium metal: it can be obtained by reducing thorium oxide with calcium, by electrolysis of anhydrous thorium chloride in a fused mixture of sodium and potassium chlorides, by calcium reduction of thorium tetrachloride mixed with anhydrous zinc chloride, and by reduction of thorium tetrachloride with an alkali metal.[14]
Reserve estimates

2012 reserve estimates:[71]
Present knowledge of the distribution of thorium resources is poor because of the relatively low-key exploration efforts arising out of insignificant demand.[72] There are two sets of estimates that define world thorium reserves, one set by the US Geological Survey (USGS) and the other supported by reports from the OECD and the International Atomic Energy Agency (the IAEA). Under the USGS estimate, USA, Australia, and India have particularly large reserves of thorium.
India and Australia are believed to possess about 300,000 tonnes each; i.e., each has 25% of the world's thorium reserves.[73] In the OECD reports, however, estimates of Australia's Reasonably Assured Reserves (RAR) of thorium indicate only 19,000 tonnes and not 300,000 tonnes as indicated by USGS. The two sources vary wildly for countries such as Brazil, Turkey, and Australia, however, both reports appear to show some consistency with respect to India's thorium reserve figures, with 290,000 tonnes (USGS) and 319,000 tonnes (OECD/IAEA).
Both the IAEA and OECD appear to conclude that India may possess the lion's share of world's thorium deposits.
The IAEA's 2005 report estimates India's reasonably assured reserves of thorium at 319,000 tonnes, but mentions recent reports of India's reserves at 650,000 tonnes.[74] A government of India estimate, shared in the country's Parliament in August 2011, puts the recoverable reserve at 846,477 tonnes.[75] The Indian Minister of State V. Narayanasamy stated that as of May 2013, the country's thorium reserves were 11.93 million tonnes (monazite, having 9-10% ThO2[71]), with a significant majority (8.59 Mt; 72%) found in the three eastern coastal states of Andhra Pradesh (3.72 Mt; 31%), Tamil Nadu (2.46 Mt; 21%) and Odisha (2.41 Mt; 20%).[76]
The prevailing estimate of the economically available thorium reserves comes from the U.S. Geological Survey, Mineral Commodity Summaries (1996–2010):[68][77]
Country | Reserves |
---|---|
Australia | 300,000 |
India | 290,000 |
Norway | 170,000 |
United States | 160,000 |
Canada | 100,000 |
South Africa | 35,000 |
Brazil | 16,000 |
Other Countries | 95,000 |
World Total | 1,200,000 |
Country | Reserves |
---|---|
India | 963,000 |
United States | 440,000 |
Australia | 300,000 |
Canada | 100,000 |
South Africa | 35,000 |
Brazil | 16,000 |
Malaysia | 4,500 |
Other Countries | 90,000 |
World Total | 1,913,000 |
Note: The OECD/NEA report notes that the estimates (that the Australian figures are based on) are subjective, due to the variability in the quality of the data, a lot of which is old and incomplete.[79] Adding to the confusion are subjective claims made by the Australian government (in 2009, through its Geoscience department) that combine the reasonably assured reserves (RAR) estimates with "inferred" data (i.e., subjective guesses). This strange combined figure of RAR and "guessed" reserves yields a figure, published by the Australian government, of 489,000 tonnes,[79] however, using the same criteria for Brazil or India would yield reserve figures of between 600,000 to 1,300,000 tonnes for Brazil and between 300,000 to 600,000 tonnes for India. Irrespective of isolated claims by the Australian government, the most credible third-party and multi-lateral reports, those of the OECD/IAEA and the USGS, consistently report high thorium reserves for India while not doing the same for Australia.
Another estimate of reasonably assured reserves (RAR) and estimated additional reserves (EAR) of thorium comes from OECD/NEA, Nuclear Energy, "Trends in Nuclear Fuel Cycle", Paris, France (2001):[80]
Country | RAR Th | EAR Th |
---|---|---|
India | 519,000 | 21% |
Australia | 489,000 | 19% |
USA | 400,000 | 13% |
Turkey | 344,000 | 11% |
Venezuela | 302,000 | 10% |
Brazil | 302,000 | 10% |
Norway | 132,000 | 4% |
Egypt | 100,000 | 3% |
Russia | 75,000 | 2% |
Greenland | 54,000 | 2% |
Canada | 44,000 | 2% |
South Africa | 18,000 | 1% |
"Other countries" | 33,000 | 2% |
"World total" | 2,810,000 |
The preceding reserve figures refer to the amount of thorium in high-concentration deposits inventoried so far and estimated to be extractable at current market prices; millions of times more total exist in Earth's 3×1019 tonne crust, around 120 trillion tons of thorium, and lesser but vast quantities of thorium exist at intermediate concentrations.[81][82][83] Proved reserves are "a poor indicator of the total future supply of a mineral resource."[83]
The Lemhi Pass, along the Idaho-Montana border, has one of the world's largest known high quality thorium deposits. Thorium Energy, Inc. has the mineral rights to approximately 1360 acres (5.5 sq km) of it and states that they have proven thorium oxide reserves of 600 thousand tons and probable reserves of an additional 1.8 million tons within their claim.[84]
In event of a thorium fuel cycle, Conway granite with 56 (±6) parts per million thorium could provide a major low-grade resource; a 307 sq mile (795 sq km) "main mass" in New Hampshire is estimated to contain over three million metric tons per 100 feet (30 m) of depth (i.e. 1 kg thorium in eight cubic metres of rock), of which two-thirds is "readily leachable".[85] Even common granite rock with 13 PPM thorium concentration (just twice the crustal average, along with 4 ppm uranium) contains potential nuclear energy equivalent to 50 times the entire rock's mass in coal,[86] although there is no incentive to resort to such very low-grade deposits so long as much higher-grade deposits remain available and cheaper to extract.[87] Thorium has been produced in excess of demand from the refining of rare earth elements.[88]
Dangers and biological roles

Powdered thorium metal is pyrophoric and often ignites spontaneously in air. Natural thorium decays very slowly compared to many other radioactive materials, and the alpha radiation emitted cannot penetrate human skin meaning owning and handling small amounts of thorium, such as a gas mantle, is considered safe. Exposure to an aerosol of thorium, however, can lead to increased risk of cancers of the lung, pancreas, and blood,[citation needed] as lungs and other internal organs can be penetrated by alpha radiation. Exposure to thorium internally leads to increased risk of liver diseases. Thorium is radioactive and produces a radioactive gas, radon-220, as one of its decay products. Secondary decay products of thorium include radium and actinium. Because of this, there are concerns about the safety of thorium mantles. Some nuclear safety agencies make recommendations about their use.[89] Production of gas mantles has led to some safety concerns during manufacture.
The element has no known biological role. Humans typically consume three micrograms per day of thorium. Of this, 99.98% does not remain in the body. Out of the thorium that does remain in the body, three quarters of it accumulates in the skeleton. A number of thorium compounds are chemically moderately toxic. People who work with thorium compounds are at a risk of dermatitis. It can take as much as thirty years after the ingestion of thorium for symptoms to manifest themselves.[16]
See also
- Accelerator-driven sub-critical reactor
- Actinides in the environment
- India's three stage nuclear power programme
- Liquid fluoride thorium reactor
- Materials science in science fiction
- Sylvania Electric Products explosion
References
- ^ "Standard Atomic Weights: Thorium". CIAAW. 2013.
- ^ Prohaska, Thomas; Irrgeher, Johanna; Benefield, Jacqueline; Böhlke, John K.; Chesson, Lesley A.; Coplen, Tyler B.; Ding, Tiping; Dunn, Philip J. H.; Gröning, Manfred; Holden, Norman E.; Meijer, Harro A. J. (4 May 2022). "Standard atomic weights of the elements 2021 (IUPAC Technical Report)". Pure and Applied Chemistry. doi:10.1515/pac-2019-0603. ISSN 1365-3075.
- ^ a b c Arblaster, John W. (2018). Selected Values of the Crystallographic Properties of Elements. Materials Park, Ohio: ASM International. ISBN 978-1-62708-155-9.
- ^ Th(-I) and U(-I) have been detected in the gas phase as octacarbonyl anions; see Chaoxian, Chi; Sudip, Pan; Jiaye, Jin; Luyan, Meng; Mingbiao, Luo; Lili, Zhao; Mingfei, Zhou; Gernot, Frenking (2019). "Octacarbonyl Ion Complexes of Actinides [An(CO)8]+/− (An=Th, U) and the Role of f Orbitals in Metal–Ligand Bonding". Chemistry (Weinheim an der Bergstrasse, Germany). 25 (50): 11772–11784. 25 (50): 11772–11784. doi:10.1002/chem.201902625. ISSN 0947-6539. PMC 6772027. PMID 31276242.
- ^ a b Greenwood, Norman N.; Earnshaw, Alan (1997). Chemistry of the Elements (2nd ed.). Butterworth-Heinemann. p. 28. ISBN 978-0-08-037941-8.
- ^ Lide, D. R., ed. (2005). "Magnetic susceptibility of the elements and inorganic compounds". CRC Handbook of Chemistry and Physics (PDF) (86th ed.). CRC Press. pp. 4–135. ISBN 978-0-8493-0486-6.
- ^ Weast, R. (1984). CRC, Handbook of Chemistry and Physics. Chemical Rubber Company Publishing. p. E110. ISBN 978-0-8493-0464-4.
- ^ Kondev, F. G.; Wang, M.; Huang, W. J.; Naimi, S.; Audi, G. (2021). "The NUBASE2020 evaluation of nuclear properties" (PDF). Chinese Physics C. 45 (3): 030001. doi:10.1088/1674-1137/abddae.
- ^ Varga, Z.; Nicholl, A.; Mayer, K. (2014). "Determination of the 229Th half-life". Physical Review C. 89 (6): 064310. doi:10.1103/PhysRevC.89.064310.
- ^ a b "IAEA-TECDOC-1450 Thorium Fuel Cycle-Potential Benefits and Challenges" (PDF). International Atomic Energy Agency. May 2005. Retrieved 15 March 2012.
- ^ Jain, S.K. "Nuclear Power – An alternative" (PDF). NPCIL. Retrieved 27 March 2012.
- ^ Bucher, R.G. (January 2009). "India's Baseline Plan for Nuclear Energy self-sufficiency stage" (PDF). Argonne National Laboratory. Retrieved 27 March 2012.
- ^ a b c Wickleder 2006, p. 61.
- ^ a b c d e f g h Hammond, C. R. (2004). The Elements, in Handbook of Chemistry and Physics 81st edition. CRC press. ISBN 0-8493-0485-7.
- ^ a b c d Hyde, Earl K. (1960). The radiochemistry of thorium (PDF). Subcommittee on Radiochemistry, National Academy of Sciences—National Research Council.
- ^ a b Emsley, John (2011). Nature's Building Blocks.
- ^ a b c "Toxicological Profile Information Sheet" (PDF). Department of Health and Human Services. Retrieved 21 May 2009.
- ^ Emsley, John (2001). Nature's Building Blocks. Oxford University Press. p. 441. ISBN 0-19-850340-7.
- ^ Dewberry, Christopher T.; Etchison, Kerry C.; Cooke, Stephen A. (2007). "The pure rotational spectrum of the actinide-containing compound thorium monoxide". Physical Chemistry Chemical Physics. 9 (35): 4895–7. Bibcode:2007PCCP....9.4895D. doi:10.1039/B709343H. PMID 17912418.
- ^ "The ACME EDM Experiment." electronedm.org
- ^ Uusitalo, J.; et al. (1995). "α decay of the new isotopes 210Th and 211Th". Phys. Rev. C. 52: 113. Bibcode:1995PhRvC..52..113U. doi:10.1103/PhysRevC.52.113.
- ^ Beck; B. R.; et al. (2007). "Energy Splitting of the Ground-State Doublet in the Nucleus 229Th". Phys. Rev. Lett. 98 (14): 142501. Bibcode:2007PhRvL..98n2501B. doi:10.1103/PhysRevLett.98.142501. PMID 17501268.
{{cite journal}}
: Unknown parameter|author-separator=
ignored (help) - ^ Avedesian, Michael M., ed. (1999). "Microstructure of Magnesium and Magnesium Alloys". Magnesium and magnesium alloys. Materials Park, OH: ASM International. p. 28. ISBN 978-0-87170-657-7.
- ^ a b c d McKetta, John J. (1996). Encyclopedia of Chemical Processing and Design: Thermoplastics to Trays, Separation, Useful Capacity. CRC Press. p. 81. ISBN 0-8247-2609-X.
- ^ a b c d e Wickleder 2006, p. 52.
- ^ Jeffus, Larry (2003). "Types of Tungsten". Welding : principles and applications. Clifton Park, N.Y.: Thomson/Delmar Learning. p. 350. ISBN 978-1-4018-1046-7.
- ^ a b c d e f Wickleder 2006, p. 53.
- ^ Thoriated Camera Lens (ca. 1970s)
- ^ Rancourt, James D. (1996). Optical thin films: user handbook. SPIE Press. p. 196. ISBN 0-8194-2285-1.
- ^ a b Bulletin of the Atomic Scientists. September/October 1997 pp. 19–20
- ^ "Historic Achievement Recognized: Shippingport Atomic Power Station, A National Engineering Historical Landmark" (PDF). p. 4. Retrieved 24 June 2006.
- ^ "Thorium Fuel for Nuclear Energy". American Scientist. Sep/October 2003.
{{cite web}}
: Check date values in:|date=
(help) - ^ "Thorium fuel cycle — Potential benefits and challenge", IAEA, May 2005
- ^ Progress on India's Thorium Nuclear Reactor and South Africa's Pebble Bed. Nextbigfuture.com (22 August 2008). Retrieved on 2011-05-01.
- ^ Nuclear Power in India|Indian Nuclear Energy. World-nuclear.org. Retrieved on 1 May 2011.
- ^ Ayhan Demirbas (2009). Biohydrogen: for future engine fuel demands. Springer. pp. 36–39. ISBN 978-1-84882-510-9.
- ^ "Thorium: Is It the Better Nuclear Fuel?", Cavendish Press, Dec 2008
- ^ Kursunoglu, Behram N., and Teller, Edward (2001). Global warming and energy policy. Kluwer Academic/Plenum Publishers. p. 4. ISBN 978-0-306-46635-9.
{{cite book}}
: CS1 maint: multiple names: authors list (link) - ^ What Is Thorium? Thorium Energy Alliance
- ^ "Date set for fuel reactor". The Telegraph (Calcutta). 2 September 2013. Retrieved 4 September 2013.
- ^ Wilson, R. (1998). "Accelerator Driven Subcritical Assemblies". Report to Energy Environment and Economy Committee, U.S. Global Strategy Council.
- ^ "Light Water Breeder Reactor:Adapting a Proven System". AtomicInsights.com. Retrieved 3 September 2009.
- ^ "Thorium". World Nuclear Association. Retrieved 3 September 2009.
- ^ Clayton, J.C. (1993). "The Shippingport Pressurized Water Reactor and Light Water Breeder Reactor" (PDF). 25. American Chemical Society meeting, Pittsburgh, PA.
- ^ Sollychin, Ray. (3 September 2009) Exploring Fuel Alternatives. Iaea.org. Retrieved on 2011-05-01.
- ^ "Thorium: Cleaner Nuclear Power?". Powertechnology.com. 10 August 2007.
- ^ "Construction of 7th Rajasthan nuclear-plant begins". IBN. 25 September 2013. Retrieved 26 October 2013.
- ^ "Development work on 300 MW advanced heavy water reactor at advanced stage". The Times Of India. 20 December 2008.
- ^ "Work on thorium-based reactor to commence soon". The Hindu. 21 February 2012.
- ^ Pham, Lisa (19 October 2009). "Considering an Alternative Fuel for Nuclear Energy". New York Times.
- ^ "Indian Thorium based reactor design complete".
- ^ "First commercial fast reactor nearly ready", The Hindu, June 29, 2012
- ^ a b Weeks, Mary Elvira (1932). "The discovery of the elements. XI. Some elements isolated with the aid of potassium and sodium: Zirconium, titanium, cerium, and thorium". Journal of Chemical Education. 9 (7): 1231. Bibcode:1932JChEd...9.1231W. doi:10.1021/ed009p1231.
- ^ Berzelius, J. J. (1829). "Untersuchung eines neues Minerals und einer darin erhalten zuvor unbekannten Erde (Investigation of a new mineral and of a previously unknown earth contained therein)". Annalen der Physik und Chemie. 16 (7): 385–415. Bibcode:1829AnP....92..385B. doi:10.1002/andp.18290920702. (modern citation: Annalen der Physik, vol. 92, no. 7, pp. 385–415)
- ^ Berzelius, J. J. (1829). "Undersökning af ett nytt mineral (Thorit), som innehåller en förut obekant jord" (Investigation of a new mineral (thorite), as contained in a previously unknown earth)". Kungliga Svenska Vetenskaps Akademiens Handlingar (Transactions of the Royal Swedish Science Academy): 1–30.
- ^ Schilling, Johannes (1902). "Die eigentlichen Thorit-Mineralien (Thorit und Orangit)". Zeitschrift für Angewandte Chemie. 15 (37): 921. doi:10.1002/ange.19020153703.
- ^ Curie, Marie (1898). "Rayons émis par les composés de l'uranium et du thorium (Rays emitted by compounds of uranium and thorium)". Comptes Rendus. 126: 1101–1103. OL 24166254M.
- ^ Schmidt, G. C. (1898). "Über die vom Thorium und den Thoriumverbindungen ausgehende Strahlung (On the radiation emitted by thorium and thorium compounds)". Verhandlungen der Physikalischen Gesellschaft zu Berlin (Proceedings of the Physical Society in Berlin). 17: 14–16.
- ^ Schmidt, G. C. (1898). "Über die von den Thorverbindungen und einigen anderen Substanzen ausgehende Strahlung (On the radiation emitted by thorium compounds and some other substances)". Annalen der Physik und Chemie. 65: 141–151. (modern citation: Annalen der Physik, vol. 301, pages 141–151 (1898)).
- ^ Simmons, John Galbraith (1996). The Scientific 100: A Ranking of the Most Influential Scientists, Past and Present. Seacaucus NJ: Carol. p. 19. ISBN 0806521392.
- ^ van Arkel, A.E. (1925). "Preparation of pure titanium, zirconium, hafnium, and thorium metal". Zeitschrift für Anorganische und Allgemeine Chemie. 148: 345–350. doi:10.1002/zaac.19251480133.
{{cite journal}}
: Unknown parameter|coauthors=
ignored (|author=
suggested) (help) - ^ Synthesis of heavy elements
- ^ Attention: This template ({{cite doi}}) is deprecated. To cite the publication identified by doi:10.1038/ngeo1205, please use {{cite journal}} (if it was published in a bona fide academic journal, otherwise {{cite report}} with
|doi=10.1038/ngeo1205
instead. - ^ Wickleder 2006, p. 55.
- ^ THORIUM Agency for Toxic Substances and Disease Registry. July 1999.
- ^ Wickleder 2006, p. 56.
- ^ "Monazite-(Ce): Monazite-(Ce) mineral information and data". Retrieved 18 May 2009.
- ^ a b "U.S. Geological Survey, Mineral Commodity Summaries – Thorium".
- ^ Lunar & Planetary Lab at The University of Arizona January 2008: Thorium Map
- ^ Crouse, David; Brown, Keith (1959). "The Amex Process for Extracting Thorium Ores with Alkyl Amines". Industrial & Engineering Chemistry. 51 (12): 1461. doi:10.1021/ie50600a030.
- ^ a b "DETAILS OF THORIUM RESERVES" (PDF). Department of Atomic Energy (India). 10 May 2012. Retrieved 12 December 2013.
- ^ Jayaram, K.M.V. "An Overview of World Thorium Resources, Incentives for Further Exploration and Forecast for Thorium Requirements in the Near Future" (PDF).
- ^ "US approves Indian nuclear deal". BBC News. 9 December 2006.
- ^ IAEA: Thorium fuel cycle — Potential benefits and challenges (PDF). p. 45.
- ^ "Availability of Thorium". Press Information Bureau, Government of India. 10 August 2011. Retrieved 27 March 2012.
- ^ Press Trust of India (14 August 2013). "Over 1.25 MT thorium reserve found in 3 years". Business Standard. Retrieved 22 August 2013.
- ^ "Information and Issue Briefs – Thorium". World Nuclear Association. Retrieved 1 November 2006.
- ^ "Status of Nuclear Power: A Global View. Y.A Sokolov 2005 IAEA" (PDF).
- ^ a b "Thorium – AIMR 2009". Australian Mines Atlas. Retrieved 1 June 2009.
- ^ IAEA: Thorium fuel cycle — Potential benefits and challenges (PDF). pp. 45(table 8), 97(ref 78).
- ^ Ragheb, M. (12 August 2011) Thorium Resources In Rare Earth Elements. scribd.com
- ^ American Geophysical Union, Fall Meeting 2007, abstract #V33A-1161. Mass and Composition of the Continental Crust
- ^ a b James D. Gwartney, Richard L. Stroup, Russell S. Sobel, David MacPherson. Economics: Private and Public Choice, 12th Edition. South-Western Cengage Learning, p. 730
- ^ Lemhi Pass Thorium. thoriumenergy.com
- ^ Adams, JA; Kline, MC; Richardson, KA; Rogers, JJ (1962). "The Conway Granite of New Hampshire As a Major Low-Grade Thorium Resource". Proceedings of the National Academy of Sciences of the United States of America. 48 (11): 1898–905. Bibcode:1962PNAS...48.1898A. doi:10.1073/pnas.48.11.1898. PMC 221093. PMID 16591014.
- ^ Hubbert, M. King Nuclear Energy and the Fossil Fuels. American Petroleum Institute Conference, 8 March 1956. Republished on 8 March 2006, by the Energy Bulletin.
- ^ Brown, Harrison (1954). The Challenge of Man's Future. New York: Viking Press.
- ^ Hedrick, James B. (1997) Thorium. US Geological Survey.
- ^ Radioactivity in Lantern Mantles. Australian Radiation Protection and Nuclear Safety Agency
Bibliography
- Wickleder, Mathias S. (2006). "Thorium". In Morss, Lester R.; Edelstein, Norman M.; Fuger, Jean (eds.). The Chemistry of the Actinide and Transactinide Elements (3rd ed.). Springer Science+Business Media. ISBN 1-4020-3555-1.
{{cite book}}
: Unknown parameter|coauthors=
ignored (|author=
suggested) (help)CS1 maint: ref duplicates default (link)
Further reading
- Martin, Richard (2012). Super Fuel: Thorium, the Green Energy Source for the Future (1st ed.). Palgrave MacMillan. p. 240. ISBN 978-0-230-11647-4 (hardback).
{{cite book}}
: Check|isbn=
value: invalid character (help) - Hargraves, Robert (25 July 2012). Thorium: Energy Cheaper than Coal. CreateSpace Independent Publishing Platform. p. 482. ISBN 978-1478161295.
External links
- International Thorium Energy Organisation – IThEO.org
- European Nuclear Society – Natural Decay Chains
- ATSDR CDC ToxFAQs: health questions about thorium
- FactSheet on Thorium, World Nuclear Association
- Thorium TV – A review of the element
- EnergyFromThorium.com – Content-rich site on Thorium as a future energy source, and its extraction technology
- TED talk by former NASA engineer Kirk Sorensen about Thorium energy production (video)
- India's experimental Thorium Fuel Cycle Nuclear Reactor (NDTV Report)
- Thorium Remix 2011 – 120 minute Creative Commons Share-Alike documentary on Thorium as an energy source
- Newspaper article about thorium power in India
- China Blazes Trail for Clean Nuclear Power from Thorium
- Thorium at The Periodic Table of Videos (University of Nottingham)
- Thorium Deposits of the United States—Energy Resources for the Future? (USGS, 2009)