User:Yinweichen/Testpage2
銣是一種化學元素,符號為Rb,原子序為37。銣是種質軟、呈銀白色的金屬,屬於鹼金屬,原子量為85.4678。單質銣的反應性極高,其性質與其他鹼金屬相似,例如會在空氣中快速氧化。自然出現的銣元素由兩種同位素組成:85Rb是唯一一種穩定同位素,佔72%;87Rb具微放射性,佔28%,其半衰期為490億年,超過宇宙年齡的三倍。
德國化學家羅伯特·威廉·本生和古斯塔夫·基爾霍夫於1861年利用當時的新技術火焰光譜法發現了銣元素。
銣化合物有不少化學和電子應用。銣金屬能夠輕易氣化,而且它有特殊的吸收光譜範圍,所以常被在原子的激光操控技術上。
銣並沒有已知的生物功用。但生物體對銣離子的處理機制和鉀離子相似,因此銣離子會被主動運輸到植物和動物細胞中。
性質
Rubidium is a very soft, ductile, silvery-white metal.[3] It is the second most electropositive of the non-radioactive alkali metals and melts at a temperature of 39.3 °C(102.7 °F). Similar to other alkali metals, rubidium metal reacts violently with water, forms amalgams with mercury and alloys with gold, iron, caesium, sodium, and potassium, but not lithium (despite the fact that rubidium and lithium are in the same group).[4] As with potassium (which is slightly less reactive) and caesium (which is slightly more reactive), rubidium's reaction with water is usually vigorous enough to ignite the hydrogen gas it liberates. Rubidium has also been reported to ignite spontaneously in air.[3] Rubidium has a very low ionization energy of only 406 kJ/mol.[5] Rubidium and potassium show a very similar purple color in the flame test, which makes spectroscopy methods necessary to distinguish the two elements.
Compounds
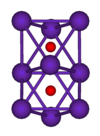
9O
2 cluster
Rubidium chloride (RbCl) is probably the most used rubidium compound; it is used in biochemistry to induce cells to take up DNA, and as a biomarker since it is readily taken up to replace potassium, and occurs in only small quantities in living organisms. Other common rubidium compounds are the corrosive rubidium hydroxide (RbOH), the starting material for most rubidium-based chemical processes; rubidium carbonate (Rb2CO3), which is used in some optical glasses, and rubidium copper sulfate, Rb2SO4·CuSO4·6H2O. Rubidium silver iodide (RbAg4I5) has the highest room temperature conductivity of any known ionic crystal, a property that is being exploited in thin film batteries and other applications.[6][7]
Rubidium has a number of oxides, including rubidium monoxide (Rb2O), Rb6O and Rb9O2, which form if rubidium metal is exposed to air; rubidium in excess oxygen gives the superoxide RbO2. Rubidium forms salts with halides, making rubidium fluoride, rubidium chloride, rubidium bromide, and rubidium iodide.
Isotopes
Although rubidium is monoisotopic, naturally occurring rubidium is composed of two isotopes: the stable 85Rb (72.2%) and the radioactive 87Rb (27.8%).[8] Natural rubidium is radioactive with specific activity of about 670 Bq/g, enough to significantly expose a photographic film in 110 days.[9][10] Aside from 85Rb and 87Rb, another 24 synthetically produced isotopes of rubidium are known, with half-lives of under 3 months; most of these are highly radioactive and have few uses.
Rubidium-87 has a half-life of ×109 years, which is more than three times the 48.8age of the universe of ±0.037)×109 years, (13.798[11] making it a primordial nuclide. It readily substitutes for potassium in minerals, and is therefore fairly widespread. Rb has been used extensively in dating rocks; 87Rb decays to stable 87Sr by emission of a negative beta particle. During fractional crystallization, Sr tends to become concentrated in plagioclase, leaving Rb in the liquid phase. Hence, the Rb/Sr ratio in residual magma may increase over time, resulting in rocks with elevated Rb/Sr ratios due to progressing differentiation. The highest ratios (10 or more) occur in pegmatites. If the initial amount of Sr is known or can be extrapolated, then the age can be determined by measurement of the Rb and Sr concentrations and of the 87Sr/86Sr ratio. The dates indicate the true age of the minerals only if the rocks have not been subsequently altered (see rubidium-strontium dating).[12][13]
Rubidium-82, one of the element's non-natural isotopes, is produced by electron-capture decay of strontium-82 with a half-life of 25.36 days. The subsequent decay of rubidium-82 with a half-life of 76 seconds to stable krypton-82 happens by positron emission.[8]
Occurrence
Rubidium is the twenty-third most abundant element in the Earth's crust, roughly as abundant as zinc and rather more common than copper.[14] It occurs naturally in the minerals leucite, pollucite, carnallite, and zinnwaldite, which contain up to 1% of its oxide. Lepidolite contains between 0.3% and 3.5% rubidium, and is the commercial source of the element.[15] Some potassium minerals and potassium chlorides also contain the element in commercially significant amounts.[16]
Seawater contains an average of 125 µg/L of rubidium compared to the much higher value for potassium of 408 mg/L and the much lower value of 0.3 µg/L for caesium.[17]
Because of its large ionic radius, rubidium is one of the "incompatible elements."[18] During magma crystallization, rubidium is concentrated together with its heavier analogue caesium in the liquid phase and crystallizes last. Therefore the largest deposits of rubidium and caesium are zone pegmatite ore bodies formed by this enrichment process. Because rubidium substitutes for potassium in the crystallization of magma, the enrichment is far less effective than in the case of caesium. Zone pegmatite ore bodies containing mineable quantities of caesium as pollucite or the lithium minerals lepidolite are also a source for rubidium as a by-product.[14]
Two notable sources of rubidium are the rich deposits of pollucite at Bernic Lake, Manitoba, Canada, and the rubicline ((Rb,K)AlSi3O8) found as impurities in pollucite on the Italian island of Elba, with a rubidium content of 17.5%.[19] Both of these deposits are also sources of caesium.
Production
Although rubidium is more abundant in Earth's crust than caesium, the limited applications and the lack of a mineral rich in rubidium limits the production of rubidium compounds to 2 to 4 tonnes per year.[14] Several methods are available for separating potassium, rubidium, and caesium. The fractional crystallization of a rubidium and caesium alum (Cs,Rb)Al(SO4)2·12H2O yields after 30 subsequent steps pure rubidium alum. Two other methods are reported, the chlorostannate process and the ferrocyanide process.[14][20]
For several years in the 1950s and 1960s, a by-product of potassium production called Alkarb was a main source for rubidium. Alkarb contained 21% rubidium, with the rest being potassium and a small fraction of caesium.[21] Today the largest producers of caesium, such as the Tanco Mine, Manitoba, Canada, produce rubidium as by-product from pollucite.[14]

History
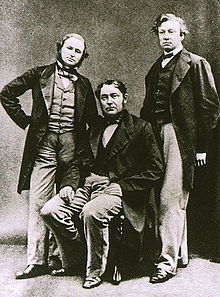
Rubidium was discovered in 1861 by Robert Bunsen and Gustav Kirchhoff, in Heidelberg, Germany, in the mineral lepidolite through the use of a spectroscope. Because of the bright red lines in its emission spectrum, they chose a name derived from the Latin word rubidus, meaning "dark red".[22][23]
Rubidium is present as a minor component in lepidolite. Kirchhoff and Bunsen processed 150 kg of a lepidolite containing only 0.24% rubidium oxide (Rb2O). Both potassium and rubidium form insoluble salts with chloroplatinic acid, but these salts show a slight difference in solubility in hot water. Therefore, the less-soluble rubidium hexachloroplatinate (Rb2PtCl6) could be obtained by fractional crystallization. After reduction of the hexachloroplatinate with hydrogen, this process yielded 0.51 grams of rubidium chloride for further studies. The first large scale isolation of caesium and rubidium compounds, performed from 44,000 liters of mineral water by Bunsen and Kirchhoff, yielded, besides 7.3 grams of caesium chloride, also 9.2 grams of rubidium chloride.[22][23] Rubidium was the second element, shortly after caesium, to be discovered spectroscopically, only one year after the invention of the spectroscope by Bunsen and Kirchhoff.[24]
The two scientists used the rubidium chloride thus obtained to estimate the atomic weight of the new element as 85.36 (the currently accepted value is 85.47).[22] They tried to generate elemental rubidium by electrolysis of molten rubidium chloride, but instead of a metal, they obtained a blue homogeneous substance which "neither under the naked eye nor under the microscope showed the slightest trace of metallic substance." They assigned it as a subchloride (Rb
2Cl); however, the product was probably a colloidal mixture of the metal and rubidium chloride.[25] In a second attempt to produce metallic rubidium, Bunsen was able to reduce rubidium by heating charred rubidium tartrate. Although the distilled rubidium was pyrophoric, it was possible to determine the density and the melting point of rubidium. The quality of the research done in the 1860s can be appraised by the fact that their determined density differs less than 0.1 g/cm3 and the melting point by less than 1 °C from the presently accepted values.[26]
The slight radioactivity of rubidium was discovered in 1908 but before the theory of isotopes was established in the 1910s and the low activity due to the long half-life of above 1010 years made interpretation complicated. The now proven decay of 87Rb to stable 87Sr through beta decay was still under discussion in the late 1940s.[27][28]
Rubidium had minimal industrial value before the 1920s.[29] Since then, the most important use of rubidium has been in research and development, primarily in chemical and electronic applications. In 1995, rubidium-87 was used to produce a Bose–Einstein condensate,[30] for which the discoverers, Eric Allin Cornell, Carl Edwin Wieman and Wolfgang Ketterle, won the 2001 Nobel Prize in Physics.[31]
Applications

Rubidium compounds are sometimes used in fireworks to give them a purple color.[32] Rubidium has also been considered for use in a thermoelectric generator using the magnetohydrodynamic principle, where rubidium ions are formed by heat at high temperature and passed through a magnetic field.[33] These conduct electricity and act like an armature of a generator thereby generating an electric current. Rubidium, particularly vaporized 87Rb, is one of the most commonly used atomic species employed for laser cooling and Bose–Einstein condensation. Its desirable features for this application include the ready availability of inexpensive diode laser light at the relevant wavelength, and the moderate temperatures required to obtain substantial vapor pressures.[34][35]
Rubidium has been used for polarizing 3He, producing volumes of magnetized 3He gas, with the nuclear spins aligned toward a particular direction in space, rather than randomly.
Rubidium vapor is optically pumped by a laser and the polarized Rb polarizes 3He through the hyperfine interaction.[36]
Such spin-polarized 3He cells are becoming popular for neutron polarization measurements and for producing polarized neutron beams for other purposes.[37]
The resonant element in atomic clocks utilizes the hyperfine structure of rubidium's energy levels, making rubidium useful for high-precision timing, and is used as the main component of secondary frequency references (rubidium oscillators) to maintain frequency accuracy in cell site transmitters and other electronic transmitting, networking, and test equipment. These rubidium standards are often used with GPS to produce a "primary frequency standard" that has greater accuracy and is less expensive than caesium standards.[38][39] Such rubidium standards are often mass-produced for the telecommunication industry.[40]
Other potential or current uses of rubidium include a working fluid in vapor turbines, as a getter in vacuum tubes, and as a photocell component.[41] Rubidium is also used as an ingredient in special types of glass, in the production of superoxide by burning in oxygen, in the study of potassium ion channels in biology, and as the vapor to make atomic magnetometers.[42] In particular, 87Rb is currently being used, with other alkali metals, in the development of spin-exchange relaxation-free (SERF) magnetometers.[42]
Rubidium-82 is used for positron emission tomography. Rubidium is very similar to potassium and therefore tissue with high potassium content will also accumulate the radioactive rubidium. One of the main uses is in myocardial perfusion imaging. The very short half-life of 76 seconds makes it necessary to produce the rubidium-82 from decay of strontium-82 close to the patient.[43] As a result of changes in the blood brain barrier in brain tumors, rubidium collects more in brain tumors than normal brain tissue, allowing the use of radioisotope rubidium-82 in nuclear medicine to locate and image brain tumors.[44]
Rubidium was tested for the influence on manic depression and depression.[45][46] Dialysis patients suffering from depression show a depletion in rubidium and therefore a supplementation may help during depression.[47] In some tests the rubidium was administered as rubidium chloride with up to 720 mg per day for 60 days.[48][49]
Precautions and biological effects
Rubidium reacts violently with water and can cause fires. To ensure safety and purity, this metal is usually kept under a dry mineral oil or sealed in glass ampoules in an inert atmosphere. Rubidium forms peroxides on exposure even to small amount of air diffusing into oil, and is thus subject to similar peroxide precautions as storage of metallic potassium.[50]
Rubidium, like sodium and potassium, almost always has +1 oxidation state when dissolved in water, including its presence in all biological systems. The human body tends to treat Rb+ ions as if they were potassium ions, and therefore concentrates rubidium in the body's intracellular fluid (i.e., inside cells).[51] The ions are not particularly toxic; a 70 kg person contains on average 0.36 g of rubidium, and an increase in this value by 50 to 100 times did not show negative effects in test persons.[52] The biological half-life of rubidium in humans was measured as 31–46 days.[45] Although a partial substitution of potassium by rubidium is possible, rats with more than 50% of their potassium substituted in the muscle tissue died.[53][54]
參考資料
- ^ Haynes, William M. CRC handbook of chemistry and physics: a ready-reference book of chemical and physical data 92nd. Boca Raton, FL.: CRC Press. 2011: 4.122. ISBN 978-1-4398-5511-9. OCLC 730008390 (英语).
- ^ Magnetic susceptibility of the elements and inorganic compounds, in Handbook of Chemistry and Physics 81st edition, CRC press.
- ^ 3.0 3.1 Ohly, Julius. Rubidium. Analysis, detection and commercial value of the rare metals. Mining Science Pub. Co. 1910.
- ^ Holleman, Arnold F.; Wiberg, Egon; Wiberg, Nils. Vergleichende Übersicht über die Gruppe der Alkalimetalle. Lehrbuch der Anorganischen Chemie 91–100. Walter de Gruyter. 1985: 953–955. ISBN 3-11-007511-3 (German).
- ^ Moore, John W; Stanitski, Conrad L; Jurs, Peter C. Principles of Chemistry: The Molecular Science. 2009: 259. ISBN 978-0-495-39079-4.
- ^ Smart, Lesley; Moore, Elaine. RbAg4I5. Solid state chemistry: an introduction. CRC Press. 1995: 176–177. ISBN 978-0-7487-4068-0.
- ^ Bradley, J. N.; Greene, P. D. Relationship of structure and ionic mobility in solid MAg4I5. Trans. Faraday Soc. 1967, 63: 2516. doi:10.1039/TF9676302516.
- ^ 8.0 8.1 Audi, Georges; Bersillon, O.; Blachot, J.; Wapstra, A.H. The NUBASE Evaluation of Nuclear and Decay Properties. Nuclear Physics A (Atomic Mass Data Center). 2003, 729 (1): 3–128. Bibcode:2003NuPhA.729....3A. doi:10.1016/j.nuclphysa.2003.11.001.
- ^ Strong, W. W. On the Possible Radioactivity of Erbium, Potassium and Rubidium. Physical Review. Series I. 1909, 29 (2): 170–173. Bibcode:1909PhRvI..29..170S. doi:10.1103/PhysRevSeriesI.29.170.
- ^ Lide, David R; Frederikse, H. P. R. CRC handbook of chemistry and physics: a ready-reference book of chemical and physical data. June 1995: 4–25. ISBN 978-0-8493-0476-7.
- ^ Planck collaboration; Ade, P. A. R.; Aghanim, N.; Armitage-Caplan, C.; Arnaud, M.; Ashdown, M.; Atrio-Barandela, F.; Aumont, J.; et al. Planck 2013 results. XVI. Cosmological parameters. Submitted to Astronomy & Astrophysics. 2013, 1303: 5076. Bibcode:2014A&A...571A..16P. arXiv:1303.5076
. doi:10.1051/0004-6361/201321591.
- ^ Attendorn, H. -G.; Bowen, Robert. Rubidium-Strontium Dating. Isotopes in the Earth Sciences. Springer. 1988: 162–165. ISBN 978-0-412-53710-3.
- ^ Walther, John Victor. Rubidium-Strontium Systematics. Essentials of geochemistry. Jones & Bartlett Learning. 1988 2009: 383–385. ISBN 978-0-7637-5922-3.
- ^ 14.0 14.1 14.2 14.3 14.4 Butterman, William C.; Brooks, William E.; Reese, Jr., Robert G. Mineral Commodity Profile: Rubidium (PDF). United States Geological Survey. 2003 [2010-12-04].
- ^ Wise, M. A. Trace element chemistry of lithium-rich micas from rare-element granitic pegmatites. Mineralogy and Petrology. 1995, 55 (13): 203–215. Bibcode:1995MinPe..55..203W. doi:10.1007/BF01162588.
- ^ Norton, J. J. Lithium, cesium, and rubidium—The rare alkali metals. Brobst, D. A., and Pratt, W. P. (编). United States mineral resources. Paper 820. U.S. Geological Survey Professional. 1973: 365–378 [2010-09-26].
- ^ Bolter, E; Turekian, K; Schutz, D. The distribution of rubidium, cesium and barium in the oceans. Geochimica et Cosmochimica Acta. 1964, 28 (9): 1459. Bibcode:1964GeCoA..28.1459B. doi:10.1016/0016-7037(64)90161-9.
- ^ McSween, Harry Y; Jr,; Huss, Gary R. Cosmochemistry. 2010: 224. ISBN 978-0-521-87862-3.
- ^ Teertstra, David K.; Cerny, Petr; Hawthorne, Frank C.; Pier, Julie; Wang, Lu-Min; Ewing, Rodney C. Rubicline, a new feldspar from San Piero in Campo, Elba, Italy. American Mineralogist. 1998, 83 (11–12 Part 1): 1335–1339.
- ^ bulletin 585. United States. Bureau of Mines. 1995.
- ^ Cesium and Rubidium Hit Market. Chemical & Engineering News. 1959, 37 (22): 50. doi:10.1021/cen-v037n022.p050.
- ^ 22.0 22.1 22.2 Kirchhoff,, G.; Bunsen, R. Chemische Analyse durch Spectralbeobachtungen. Annalen der Physik und Chemie. 1861, 189 (7): 337–381. Bibcode:1861AnP...189..337K. doi:10.1002/andp.18611890702.
- ^ 23.0 23.1 Weeks, Mary Elvira. The discovery of the elements. XIII. Some spectroscopic discoveries. Journal of Chemical Education. 1932, 9 (8): 1413–1434. Bibcode:1932JChEd...9.1413W. doi:10.1021/ed009p1413.
- ^ Ritter, Stephen K. C&EN: It's Elemental: The Periodic Table – Cesium. American Chemical Society. 2003 [2010-02-25].
- ^ Zsigmondy, Richard. Colloids and the Ultra Microscope. Read books. 2007: 69 [2010-09-26]. ISBN 978-1-4067-5938-9.
- ^ Bunsen, R. Ueber die Darstellung und die Eigenschaften des Rubidiums. Annalen der Chemie und Pharmacie. 1863, 125 (3): 367. doi:10.1002/jlac.18631250314.
- ^ Lewis, G.M. The natural radioactivity of rubidium. Philosophical Magazine Series 7. 1952, 43 (345): 1070–1074. doi:10.1080/14786441008520248.
- ^ Campbell, N. R.; Wood, A. The Radioactivity of Rubidium. Proceedings of the Cambridge Philosophical Society. 1908, 14: 15.
- ^ Butterman, W.C.; Reese, Jr., R.G. Mineral Commodity Profiles Rubidium (PDF). United States Geological Survey. [2010-10-13].
- ^ Press Release: The 2001 Nobel Prize in Physics. [2010-02-01].
- ^ Levi, Barbara Goss. Cornell, Ketterle, and Wieman Share Nobel Prize for Bose-Einstein Condensates. Physics Today (Physics Today online year = 2001): 14. Bibcode:2001PhT....54l..14L. doi:10.1063/1.1445529.
- ^ Koch, E.-C. Special Materials in Pyrotechnics, Part II: Application of Caesium and Rubidium Compounds in Pyrotechnics. Journal Pyrotechnics. 2002, 15: 9–24.
- ^ Boikess, Robert S; Edelson, Edward. Chemical principles. 1981: 193. ISBN 978-0-06-040808-4.
- ^ Eric Cornell; et al. Bose-Einstein condensation (all 20 articles) 101. 1996: 419–618. doi:10.6028/jres.101.045.
|journal=
被忽略 (帮助);|issue=
被忽略 (帮助) - ^ Martin, J L; McKenzie, C R; Thomas, N R; Sharpe, J C; Warrington, D M; Manson, P J; Sandle, W J; Wilson, A C. Output coupling of a Bose-Einstein condensate formed in a TOP trap. Journal of Physics B: Atomic, Molecular and Optical Physics. 1999, 32 (12): 3065. Bibcode:1999JPhB...32.3065M. arXiv:cond-mat/9904007
. doi:10.1088/0953-4075/32/12/322.
- ^ Gentile, T. R.; Chen, W. C.; Jones, G. L.; Babcock, E.; Walker, T. G. Polarized 3He spin filters for slow neutron physics (PDF). Journal of Research of the National Institute of Standards and Technology: 299–304.
- ^ Neutron spin filters based on polarized helium-3. NIST Center for Neutron Research 2002 Annual Report. [2008-01-11].
- ^ Eidson, John C. GPS. Measurement, control, and communication using IEEE 1588. 2006-04-11: 32. ISBN 978-1-84628-250-8.
- ^ King, Tim; Newson, Dave. Rubidium and crystal oscillators. Data network engineering. 1999-07-31: 300. ISBN 978-0-7923-8594-3.
- ^ Marton, L. Rubidium Vapor Cell. Advances in electronics and electron physics. 1977-01-01. ISBN 978-0-12-014644-4.
- ^ Mittal. Introduction To Nuclear And Particle Physics. 2009: 274. ISBN 978-81-203-3610-0.
- ^ 42.0 42.1 Li, Zhimin; Wakai, Ronald T.; Walker, Thad G. Parametric modulation of an atomic magnetometer. Applied Physics Letters. 2006, 89 (13): 134105. Bibcode:2006ApPhL..89m4105L. doi:10.1063/1.2357553.
- ^ Jadvar, H.; Anthony Parker, J. Rubidium-82. Clinical PET and PET/CT. 2005: 59. ISBN 978-1-85233-838-1.
- ^ Yen, CK; Yano, Y; Budinger, TF; Friedland, RP; Derenzo, SE; Huesman, RH; O'Brien, HA. Brain tumor evaluation using Rb-82 and positron emission tomography. Journal of Nuclear Medicine. 1982, 23 (6): 532–7. PMID 6281406.
- ^ 45.0 45.1 Paschalis, C; Jenner, F A; Lee, C R. Effects of rubidium chloride on the course of manic-depressive illness. J R Soc Med. 1978, 71 (9): 343–352. PMC 1436619
. PMID 349155.
- ^ Malekahmadi, P; Williams, John A. Rubidium in psychiatry: Research implications. Pharmacology Biochemistry and Behavior. 1984, 21: 49. doi:10.1016/0091-3057(84)90162-X.
- ^ Canavese, Caterina; Decostanzi, Ester; Branciforte, Lino; Caropreso, Antonio; Nonnato, Antonello; Sabbioni, Enrico. Depression in dialysis patients: Rubidium supplementation before other drugs and encouragement?. Kidney International. 2001, 60 (3): 1201–1201. doi:10.1046/j.1523-1755.2001.0600031201.x.
- ^ Lake, James A. Textbook of Integrative Mental Health Care. New York: Thieme Medical Publishers. 2006: 164–165. ISBN 1-58890-299-4.
- ^ Torta, R; Ala, G; Borio, R; Cicolin, A; Costamagna, S; Fiori, L; Ravizza, L. Rubidium chloride in the treatment of major depression. Minerva psichiatrica. 1993, 34 (2): 101–10. PMID 8412574.
- ^ Martel, Bernard; Cassidy, Keith. Rubidium. Chemical risk analysis: a practical handbook. 2004-07-01: 215. ISBN 978-1-903996-65-2.
- ^ Relman, AS. The Physiological Behavior of Rubidium and Cesium in Relation to That of Potassium. The Yale journal of biology and medicine. 1956, 29 (3): 248–62. PMC 2603856
. PMID 13409924.
- ^ Fieve, Ronald R.; Meltzer, Herbert L.; Taylor, Reginald M. Rubidium chloride ingestion by volunteer subjects: Initial experience. Psychopharmacologia. 1971, 20 (4): 307–14. PMID 5561654. doi:10.1007/BF00403562.
- ^ Meltzer, HL. A pharmacokinetic analysis of long-term administration of rubidium chloride. Journal of clinical pharmacology. 1991, 31 (2): 179–84. PMID 2010564. doi:10.1002/j.1552-4604.1991.tb03704.x.
- ^ Follis, Richard H., Jr. Histological Effects in rats resulting from adding Rubidium or Cesium to a diet deficient in potassium. AJP – Legacy. 1943, 138 (2): 246.
延伸閱讀
- Meites, Louis (1963). Handbook of Analytical Chemistry (New York: McGraw-Hill Book Company, 1963)
- Steck, Daniel A. Rubidium-87 D Line Data (PDF). Los Alamos National Laboratory (technical report LA-UR-03-8638).
外部鏈接
- Rubidium at The Periodic Table of Videos (University of Nottingham)