User:BioMCdx/sandbox
Complex I mitochondrial respiratory chain deficiency, also known as mitochondrial complex I deficiency, is a clinical condition where mitochondrial complex I, an essential protein for energy production in mitochondria, is in shortage.[1] Disorders related to malfunctioning mitochondria are one of the most common genetic disorders where the prevalence is 12.5 out of 100,000, and among those mitochondrial disorders, mitochondrial complex I deficiency is the most common type.[2][3] A distinguishing characteristic of mitochondrial complex I deficiency is that the symptoms are multi-systemic and widely varied as mitochondria are present in every human cell.[4] To be specific, this disease mainly affects the brain, the heart, the muscle, and the eye as those organs require a large energy supply for their functions.[2] In addition, the blood is also affected because excessive metabolites from malfunctioning mitochondria accumulate in it.[5] Although symptoms differ from patient to patient, the impairment of oxidative phosphorylation, the major process for energy production and metabolism, is the basic mechanism of the disease, since mitochondrial complex I is crucial in oxidative phosphorylation.[6][7]
Specifically, mitochondrial complex I deficiency is caused either by the mutation in nuclear DNA (nDNA) or by the mutation in mitochondrial DNA (mtDNA) that encode for the complex I or other assembly factors.[8] By utilizing this characteristic, a diagnosis can be performed with genetic tests.[2] However, since genotype-phenotype is not yet fully understood, enzymatic function tests and metabolite analyses are also integrated for a precise diagnosis.[9] Nevertheless, even with an early, accurate diagnosis, patients cannot be completely cured because there is no treatment for the cause of the disease.[10] Fortunately, medications, dietary interventions, and exercise therapies can be prescribed to deal with diverse symptoms of the disease.[11][12] Apart from this, novel, direct cures for this disease are in a developmental stage, which is gene therapy and mitochondrial replacement therapy.[11][13]
Signs & symptoms
[edit]
In mitochondrial complex I deficiency, the symptoms can manifest in any tissues or organs, but organs with high energy demand are predominantly affected.[14] Specifically, Leigh syndrome manifests in over 80% of the patients, and hypotonia manifests in over 60% of the patients.[10] Additionally, the patients may also show a wide variety of symptoms such as cardiomyopathies, Leber's hereditary optic neuropathy (LHON), and lactic acidosis.[5][10]
Brain
[edit]Leigh syndrome is a neurological disease in which basal nuclei, midbrain and medulla oblongata are destructed bilaterally.[15] This syndrome appears in most patients within eight months from the initial onset of the disease, and the main symptoms are developmental delay in brain functions (e.g. speech and cognitive function), hypotonia, dystonia, ataxia.[15][16] Besides Leigh syndrome, various neurological conditions can manifest, including general and focal seizures, migraine, and dementia.[17][18]
Muscle
[edit]Due to deficiency of mitochondrial complex I, muscle tissues in various parts of the body receive insufficient energy supply, which causes myopathy. The main symptoms of myopathy are muscle weakness and exercise intolerance, and reduced muscle activity of various muscles can lead to other severe conditions.[13] For example, weakness in oropharyngeal muscles can impair swallowing, and weakness in thoracic muscles can cause shortness of breath. Furthermore, limb muscle weakness causes reduced mobility, and eye muscle and upper eyelid muscle weakness cause double vision and drooping eyelids.[18] Moreover, the patients who suffer from exercise intolerance may easily experience fatigue.[18]
Heart
[edit]Cardiomyopathy, the stiffened cardiac muscle, is another common clinical manifestation of mitochondrial complex I deficiency.[19] Patients with cardiomyopathy can either be asymptomatic or symptomatic where irregular heart rhythm, heart failure, or even cardiac arrest may occur.[19] The most common type of cardiomyopathy observed in complex I deficiency patients is hypertrophic cardiomyopathy, where the heart muscle is enlarged which partially blocks the blood flow in the heart chamber.[10] The common symptoms observed in this condition are chest pain, fainting, shortness of breath, and tachycardia (fast heart rate).[19] Patients with mutation in NDUFS2, NDUFS8, NDUFA2, NDUFS4 are most frequently affected by hypertrophic cardiomyopathy.[10]
Eye
[edit]For the symptoms in the eye, LHON is the most common symptom.[20] LHON is neurodegeneration which results in rapid loss of retinal ganglion cell that are responsible for sending visual information to the brain.[20] From the onset of this syndrome, optic atrophy, which means retinal nerve fiber damage, occurs dynamically for 12 months, which leads to impaired vision.[20] For the patients suffering from LHON, the usual symptom reported is a color vision impairment, but the pupillary reflex is not affected, and they feel no pain during the degeneration.[21]
Blood
[edit]For patients suffering from complex I deficiency, it is common to manifest lactic acidosis, which is a clinical condition where excessive lactic acid in the bloodstream causes a decrease in blood pH level.[22] Such a condition is triggered because complex I deficiency leads to the accumulation of pyruvate, which is converted into lactate.[22] For the patients with lactic acidosis, reduction in contractility of the heart, arrhythmia, and kidney diseases can occur.[23]
Causes
[edit]
Causes of mitochondrial complex I deficiency can be classified into two categories: primary and secondary causes.[10] The primary cause is the defect in 44 genes encoding subunits of mitochondrial complex I and its assembly factors functioning for the assembly of the subunits into mitochondrial complex I.[10] The secondary cause is the defect in other auxiliary genes such as the genes encoding for Fe-S Cluster assembly and the genes encoding for ubiquinone.[10] The cause-and-effect relationship for the primary cause is clear from intensive research, while the relationship is not yet well established for the secondary cause.[10]
In detail, mitochondrial complex I is composed of 45 subunits consisting of 38 encoded in nDNA and 7 encoded in mtDNA.[2] There are two types of subunits: the core subunit, which is involved in fundamental roles, and the supernumerary subunit, which assists in the function of core subunits.[2] There are 7 core subunits encoded by nDNA: NDUFS1, NDUFS2, NDUFS3, NDUFS7, NDUFS8, NDUFV1, and NDUFV2.[2] Besides that, all subunits encoded by mtDNA are core subunits: MTND1, MTND2, MTND3, MTND4, MTND4L, MTND5, and MTND6.[2]
Among 38 subunits encoded by nDNA, 21 subunits, including 7 core subunits, are found to have mutations in the patients and each core subunit encoded by mtDNA also has mutations causing the disease.[10] Furthermore, since core subunits are essential for mitochondrial complex I, a missense mutation, a single change of amino acid, can lead to clinical diseases, and a null-type mutation, which causes the failure of translation of the protein, can be fatal.[2] Thus, in the patients' DNA, a missense mutation is predominant in core subunit genes, whereas a null-type mutation is predominant in supernumerary subunit genes.[2] On the other hand, assembly factors have no defined core protein, but a high proportion of missense mutations can reveal what plays fundamental roles.[2]
Mechanism of OXPHOS
[edit]
Mitochondrial complex I is involved in oxidative phosphorylation (OXPHOS) as one of the 5 complexes.[24] OXPHOS is a process of producing ATP, which is a universal energy source for human cells.[7][24] To be specific, complex I oxidizes NADH and obtains two electrons that can be used to reduce ubiquinone to ubiquinol.[7] This molecule is then oxidized at the complex III, which in turn reduces cytochrome c.[7] Finally, cytochrome c can transfer electrons to the complex IV that can reduce oxygen into water molecules and pump out protons into the intermembrane space.[25] When the proton gradient is built up, protons tend to flow back to the mitochondrial matrix following the gradient, and complex V utilizes such force to drive the conversion from ADP to ATP.[25] In complex I deficiency, not enough electrons can be delivered to complex IV for the proton pumping, so the capability of the cell to produce energy is significantly impaired.[25]
Diagnosis
[edit]Diagnosis of mitochondrial complex I deficiency requires a multi-disciplinary approach due to its wide range of symptoms and the poor correlation between genotype and phenotype.[4] Diagnosis is progressed with three main analyses: metabolite analysis, biochemical analysis, and genetic analysis.[4]

Metabolite analysis
[edit]Metabolite analysis is performed with blood and urine samples to detect significant signs of the disease such as increased lactate to pyruvate ratio.[9] Moreover, an increased amount of ketone body such as beta-hydroxybutyric acid and diacetic acid is another notable sign of the disease.[9] Nevertheless, since such features can be observed in a variety of diseases other than mitochondrial complex I deficiency, metabolic indicators cannot solely confirm the presence of the disease.[9]
Biochemical analysis
[edit]Biochemical analysis is usually conducted with tissue samples obtained from muscle biopsy, and enzyme activities of the OXPHOS system can be measured through the analysis.[9] Even though gel assay was traditionally utilized to test the enzyme activities, nowadays, spectrophotometry is used for accurate, quantitative analysis and precise detection of mild deficiencies.[9] The main signs that indicate the complex I deficiency are the reduction of mitochondrial complex I activity and the elevation of citrate synthase activity.[9] Nonetheless, since the positive and the negative results are in a narrow range, a definite diagnosis can only be made with other analyses.[9]
Genetic analysis
[edit]Mitochondrial complex I deficiency can be diagnosed by denaturing high performance liquid chromatography (DHPLC), which scans DNA and detects mutations.[2] DHPLC is time and cost-efficient as this technique selectively scans specific genes that are highly correlated with the disease.[2] Massive parallel sequencing is another recent technique for diagnosis, which is capable of sequencing a genome rapidly and cost-efficiently.[2] This technique does not require prior knowledge of gene function and hence can detect rarely seen mutations.[2]
Treatment
[edit]Until now, there is no cure for mitochondrial complex I deficiency, but there are some treatments that can relieve the symptoms of the patients.[11][12] The treatment options available for the patients are exercise therapy, medication, and dietary intervention.[11][12][13]
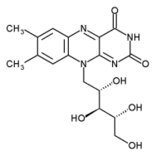
Physical therapy
[edit]Because one of the key features of this disease is weakened muscle, it is important to prescribe exercise for the patients to provide endurance training which can help their muscle cells to become more efficient in energy production.[11] The research shows that muscle training can increase the number of mitochondria and the efficiency of oxidative phosphorylation, thus the training can increase the efficacy of ATP production of muscle cells.[11]
Medication
[edit]For medication, biomolecules that can help out cells to bypass the complex I or enhance the efficacy of complex I are applied.[12] Riboflavin is efficient in increasing the efficiency of complex I, and succinate can directly donate electrons to FAD, which can bypass complex I and deliver electrons directly to complex III for oxidative phosphorylation.[12] Lastly, idebenone is administered, because it can also bypass complex I.[12] Specifically, idebenone showed high effectiveness in curing LHON.[11]
Dietary intervention
[edit]
For the patients, a ketogenic diet, which consists of high fat, moderate protein, and low carbohydrate diet, is recommended.[11][26] This is beneficial for the patients as it can increase the efficiency of mitochondrial function and the mitochondrial size, which leads to elevation of mitochondrial activities.[11][26] Hence, through this diet, oxidative phosphorylation efficiency will also increase.[11]
Potentially promising therapy
[edit]There are some therapies aiming to eradicate the disease by dealing with the main cause of complex I deficiency, which is a genetic mutation in the nDNA or mtDNA.[11][13] Promising gene therapy is suggested with the use of CRISPR-Cas9 technology which can precisely cut the mutated genes out and insert wild-type genes.[11] Furthermore, to treat patients with mtDNA mutations, mitochondrial replacement therapy is being developed, which extracts maternal nDNA and injects it into an oocyte with normal mitochondria.[13]
Epidemiology
[edit]Unfortunately, so far there is no established research regarding the global prevalence rate of mitochondrial diseases.[3] Nevertheless, from several regional studies, it can be estimated that 12.5 in 100,000 people suffer from any type of mitochondrial disorder.[3] Among these diseases, mitochondrial complex I deficiency is the most common disease which takes up about one-third of the total cases.[2]
Furthermore, although the age of onset for this disease varies significantly, the median age of onset is 10 months for those with mtDNA mutations and 3 months for those with nDNA mutations.[5] Moreover, three-quarters of patients die before reaching the age of ten years, and half of the patients die before reaching the age of two years.[10]
References
[edit]- ^ Pagniez-Mammeri, Hélène; Loublier, Sandrine; Legrand, Alain; Bénit, Paule; Rustin, Pierre; Slama, Abdelhamid (2012-02-01). "Mitochondrial complex I deficiency of nuclear origin: I. Structural genes". Molecular Genetics and Metabolism. 105 (2): 163–172. doi:10.1016/j.ymgme.2011.11.188. ISSN 1096-7192.
- ^ a b c d e f g h i j k l m n o Tucker, Elena J.; Compton, Alison G.; Calvo, Sarah E.; Thorburn, David R. (2011). "The molecular basis of human complex I deficiency". IUBMB Life: n/a–n/a. doi:10.1002/iub.495. ISSN 1521-6543.
- ^ a b c Schaefer, Andrew; Lim, Albert; Gorman, Grainne (2019), "Epidemiology of Mitochondrial Disease", Diagnosis and Management of Mitochondrial Disorders, Cham: Springer International Publishing, pp. 63–79, ISBN 978-3-030-05516-5, retrieved 2021-04-01
- ^ a b c Khan, Nahid Akhtar; Govindaraj, Periyasamy; Meena, Angamuthu Kannan; Thangaraj, Kumarasamy (January 2015). "Mitochondrial disorders: challenges in diagnosis & treatment". The Indian Journal of Medical Research. 141 (1): 13–26. doi:10.4103/0971-5916.154489. ISSN 0971-5916. PMC 4405934. PMID 25857492.
{{cite journal}}
: CS1 maint: unflagged free DOI (link) - ^ a b c Swalwell, Helen; Kirby, Denise M; Blakely, Emma L; Mitchell, Anna; Salemi, Renato; Sugiana, Canny; Compton, Alison G; Tucker, Elena J; Ke, Bi-Xia; Lamont, Phillipa J; Turnbull, Douglass M (July 2011). "Respiratory chain complex I deficiency caused by mitochondrial DNA mutations". European Journal of Human Genetics. 19 (7): 769–775. doi:10.1038/ejhg.2011.18. ISSN 1018-4813. PMC 3137493. PMID 21364701.
- ^ Hirst, Judy (2013-06-02). "Mitochondrial Complex I". Annual Review of Biochemistry. 82 (1): 551–575. doi:10.1146/annurev-biochem-070511-103700. ISSN 0066-4154.
- ^ a b c d Sharma, Lokendra K.; Lu, Jianxin; Bai, Yidong (2009). "Mitochondrial Respiratory Complex I: Structure, Function and Implication in Human Diseases". Current medicinal chemistry. 16 (10): 1266–1277. ISSN 0929-8673. PMC 4706149. PMID 19355884.
- ^ Alston, Charlotte L; Rocha, Mariana C; Lax, Nichola Z; Turnbull, Doug M; Taylor, Robert W (January 2017). "The genetics and pathology of mitochondrial disease: Mitochondrial genetic disease". The Journal of Pathology. 241 (2): 236–250. doi:10.1002/path.4809. PMC 5215404. PMID 27659608.
{{cite journal}}
: CS1 maint: PMC format (link) - ^ a b c d e f g h Rodenburg, Richard J. T. (April 2011). "Biochemical diagnosis of mitochondrial disorders". Journal of Inherited Metabolic Disease. 34 (2): 283–292. doi:10.1007/s10545-010-9081-y. ISSN 0141-8955. PMC 3063578. PMID 20440652.
- ^ a b c d e f g h i j k Rodenburg, Richard J. (July 2016). "Mitochondrial complex I-linked disease". Biochimica et Biophysica Acta (BBA) - Bioenergetics. 1857 (7): 938–945. doi:10.1016/j.bbabio.2016.02.012. ISSN 0005-2728.
- ^ a b c d e f g h i j k l Koňaříková, E; Marković, A; Korandová, Z; Houštěk, J; Mráček, T (2020-12-22). "Current progress in the therapeutic options for mitochondrial disorders". Physiological Research: 967–994. doi:10.33549/physiolres.934529. ISSN 1802-9973.
- ^ a b c d e f Avula, Sreenivas; Parikh, Sumit; Demarest, Scott; Kurz, Jonathan; Gropman, Andrea (June 2014). "Treatment of Mitochondrial Disorders". Current Treatment Options in Neurology. 16 (6): 292. doi:10.1007/s11940-014-0292-7. ISSN 1092-8480. PMC 4067597. PMID 24700433.
{{cite journal}}
: CS1 maint: PMC format (link) - ^ a b c d e De Vivo, Darryl C. & DiMauro, Salvatore (2017). "42 - Mitochondrial diseases". In Swaiman, K. F.; et al. (ed.).Swaiman's pediatric neurology : principles and practice (6th ed.). Edinburgh: Elsevier. pp. 334–346. ISBN 978-0-323-37481-1. OCLC 988768908.
- ^ Irwin, Michael H.; Parameshwaran, Kodeeswaran; Pinkert, Carl A. (January 2013). "Mouse models of mitochondrial complex I dysfunction". The International Journal of Biochemistry & Cell Biology. 45 (1): 34–40. doi:10.1016/j.biocel.2012.08.009. ISSN 1878-5875. PMC 3508304. PMID 22903069.
- ^ a b Lim, Albert Zishen; McFarland, Robert (2019), "Leigh Syndrome", Diagnosis and Management of Mitochondrial Disorders, Cham: Springer International Publishing, pp. 151–167, ISBN 978-3-030-05516-5, retrieved 2021-04-01
- ^ Lake, Nicole J.; Bird, Matthew J.; Isohanni, Pirjo; Paetau, Anders (June 2015). "Leigh Syndrome". Journal of Neuropathology & Experimental Neurology. 74 (6): 482–492. doi:10.1097/nen.0000000000000195. ISSN 0022-3069.
- ^ Finsterer, Josef; Mahjoub, Sinda Zarrouk (2012-06-01). "Epilepsy in mitochondrial disorders". Seizure - European Journal of Epilepsy. 21 (5): 316–321. doi:10.1016/j.seizure.2012.03.003. ISSN 1059-1311. PMID 22459315.
- ^ a b c Hirano, Michio; Emmanuele, Valentina; Quinzii, Catarina M. (2011-09-06), "Mitochondrial Myopathies", Neuromuscular Disorders, Oxford, UK: Wiley-Blackwell, pp. 42–50, ISBN 978-1-119-97333-1, retrieved 2021-04-01
- ^ a b c El-Hattab, Ayman W.; Scaglia, Fernando (2016). "Mitochondrial Cardiomyopathies". Frontiers in Cardiovascular Medicine. 3: 25. doi:10.3389/fcvm.2016.00025. ISSN 2297-055X. PMC 4958622. PMID 27504452.
{{cite journal}}
: CS1 maint: unflagged free DOI (link) - ^ a b c Carelli, Valerio; La Morgia, Chiara; Klopstock, Thomas (2019), "Mitochondrial Optic Neuropathies", Diagnosis and Management of Mitochondrial Disorders, Cham: Springer International Publishing, pp. 125–139, ISBN 978-3-030-05516-5, retrieved 2021-04-01
- ^ Yu-Wai-Man, P.; Turnbull, D. M.; Chinnery, P. F. (March 2002). "Leber hereditary optic neuropathy". Journal of Medical Genetics. 39 (3): 162–169. doi:10.1136/jmg.39.3.162. ISSN 1468-6244. PMC 1735056. PMID 11897814.
- ^ a b Grad, Leslie I.; Sayles, Leanne C.; Lemire, Bernard D. (2005-12-20). "Introduction of an additional pathway for lactate oxidation in the treatment of lactic acidosis and mitochondrial dysfunction in Caenorhabditis elegans". Proceedings of the National Academy of Sciences of the United States of America. 102 (51): 18367–18372. doi:10.1073/pnas.0506939102. ISSN 0027-8424. PMC 1311736. PMID 16344465.
- ^ Kraut, Jeffrey A.; Madias, Nicolaos E. (2010-03-23). "Metabolic acidosis: pathophysiology, diagnosis and management". Nature Reviews Nephrology. 6 (5): 274–285. doi:10.1038/nrneph.2010.33. ISSN 1759-5061.
- ^ a b Signes, Alba; Fernandez-Vizarra, Erika (2018-07-20). Garone, Caterina; Minczuk, Michal (eds.). "Assembly of mammalian oxidative phosphorylation complexes I–V and supercomplexes". Essays in Biochemistry. 62 (3): 255–270. doi:10.1042/EBC20170098. ISSN 0071-1365. PMC 6056720. PMID 30030361.
{{cite journal}}
: CS1 maint: PMC format (link) - ^ a b c Tarnopolsky, Mark; Alshahoumi, Rashid (2016-01-01), Saneto, Russell P.; Parikh, Sumit; Cohen, Bruce H. (eds.), "Chapter 29 - Complex I Deficiency", Mitochondrial Case Studies, Boston: Academic Press, pp. 257–264, doi:10.1016/b978-0-12-800877-5.00029-2, ISBN 978-0-12-800877-5, retrieved 2021-04-01
- ^ a b Branco, Ana F.; Ferreira, André; Simões, Rui F.; Magalhães-Novais, Sílvia; Zehowski, Cheryl; Cope, Elisabeth; Silva, Ana Marta; Pereira, Daniela; Sardão, Vilma A.; Cunha-Oliveira, Teresa (2016-03-XX). "Ketogenic diets: from cancer to mitochondrial diseases and beyond". European Journal of Clinical Investigation. 46 (3): 285–298. doi:10.1111/eci.12591.
{{cite journal}}
: Check date values in:|date=
(help)